ORIGINAL RESEARCH article
Gender-based performance in mathematical facts and calculations in two elementary school samples from chile and spain: an exploratory study.
- 1 Departamento de Psicología Evolutiva y de la Educación, Facultad de Educación, Economía y Tecnología de Ceuta, Universidad de Granada, Ceuta, Spain
- 2 Instituto de Estudios Sociales y Humanísticos, Facultad de Ciencias Sociales y Humanidades, Universidad Autónoma de Chile, Región Metropolitana, Chile
- 3 Departamento de Psicología Experimental, Universidad de Sevilla, Sevilla, Spain
- 4 Departamento de Psicología, Universidad Autónoma de Chile, Santiago, Chile
- 5 Núcleo Científico y Tecnológico en Ciencias Sociales y Humanidades, Universidad de la Frontera, Temuco, Chile
- 6 Escuela de Trabajo Social, Facultad de Ciencias Sociales, Universidad de Valparaíso, Valparaíso, Chile
- 7 Departamento de Psicología, Universidad Loyola Andalucía, Sevilla, Spain
Gender differences in mathematical performance are not conclusive according to the scientific literature, although such differences are supported by international studies such as the Trends in International Mathematics and Science Study (TIMSS). According to TIMSS 2019, fourth-grade male students outperformed female students in Spanish-speaking countries, among others. This work approaches the study on gender difference by examining the basic calculation skills needed to handle more complex problems. Two international samples of second and third graders from Chile and Spain were selected for this exploratory study. Tests on basic mathematical knowledge (symbolic and non-symbolic magnitude comparisons, fluency, and calculation) were administered. The tests did not show significant difference or size effect between genders for mean performance, variance in the distribution of performance, or percentiles. As noted in the existing literature on this topic and reiterated by these findings, great care should be exercised when reporting on possible gender differences in mathematical performance, as these can contribute to low self-concept among female students.

Introduction
As technology grows by leaps and bounds, new fields of study and analysis tools are also expanding: Big Data, artificial intelligence, modeling engineering, software architecture, etc. This has increased the demand for professionals, both men and women, with the solid knowledge of mathematics needed for such roles ( Belloum et al., 2019 ). However, women continue to be underrepresented in this kind of professional roles but overrepresented in lower paying jobs ( Adams et al., 2019 ). Determining the origin of these differences is challenging as cognitive, social, and cultural factors over the course of one's life may all contribute, making it difficult to connect mathematical abilities during childhood to gender differences on the job market. However, if this gender gap were intrinsic, it could appear during childhood, making it essential to determine whether this is the case and, thus, intervene to avoid future underrepresentation of women.
The reduced presence of women in mathematical fields is nothing new. As indicated by Kane and Mertz (2012 , p. 10), differences between males and females have been found in the mathematics participation rate, mean and high-end performance, and variance in the distribution of performance, making gender difference the subject of extensive debate. Havelock Ellis (1894) was the first to intend to empirically justify the differences between men and women with his variability hypothesis. According to this hypothesis, men could be expected to show greater variability than women in physical and intellectual abilities. This would explain the predominance of men in higher academic and scientific positions, but also their prevalence in crime. This hypothesis coincided with the expanded presence of women in higher education and their demands for recognition ( Shields, 1982 ) and continued to be defended, with minor modifications, until the 21st century, when the focus turned to extreme scores and percentiles. In particular, some studies found more females in the lower percentiles and more males in the higher percentiles for different math assignments ( Barbaresi et al., 2005 ; Reigosa-Crespo et al., 2012 ; Anaya et al., 2017 ).
Nevertheless, in a wide study carried out by Baker and Jones (1993) with 77,602 students from 19 countries, mathematical performance between genders was found to vary by country and the type of education students received; additionally, the differences were noted to be decreasing over time. The authors also largely debunked the hypothesis of variability when they pointed out that gender differences in performance decrease when women have more equitable access to higher education and qualified work. Indeed, several studies (for instance, Kane and Mertz, 2012 ) have moved away from the deterministic hypothesis of variability, providing empirical evidence for a hypothesis of sociocultural factors.
Coinciding with the argument that sociocultural factors have the greatest bearing on mathematical performance, differences between genders should gradually disappear as society becomes more progressive and inclusive. Lindberg et al. (2010) support this hypothesis in a broad meta-analysis. Based on 242 studies published between 1999 and 2007 (a total sample of 1,286,350 individuals both males and females), the authors concluded that mathematical performance is similar for both genders, noting that any gender difference can be attributed to unrelated factors. The authors conclude that it is crucial to disseminate these results to counteract stereotypes about [alleged] female math inferiority ( Lindberg et al., 2010 , p. 1134). More recent studies have replicated these results (for instance, Voyer and Voyer, 2014 ; Scheiber et al., 2015 ; Kersey and Cantlon, 2018 ), and others have limited the differences to a minority of tasks ( Hutchison et al., 2019 ).
Given the empirical evidence reported in the literature, males and females would be expected to perform similarly on international studies such as the TIMSS ( Instituto Nacional de Evaluación Educativa [INEE], 2016 , 2020 ). Unfortunately, this is not the case. According to the TIMSS, the gender gap in mathematical performance is present as early as fourth grade and, according to the last edition (2019), has even increased in countries such as Spain. Gender differences at the fourth grade level can also be noted, although to a lesser extent, in other Spanish-speaking countries that participated in TIMSS 2019. Chile, for example, went from having no significant gender differences in mathematics in the 2015 report to having significant ones in the 2019 report ( Instituto Nacional de Evaluación Educativa [INEE], 2016 , 2020 ). In fact, samples from Spanish-speaking countries show a large gender gap in international reports, but few studies include them in their analysis (e.g., the meta-analysis by Voyer and Voyer, 2014 ).
This exploratory study considers two Spanish-speaking samples from Chile and Spain, both of which are underrepresented in the literature. First, it is important to analyze potential gender differences for these countries. Second, by looking into the samples from these two countries, common patterns may be found that could allow an early intervention to be designed.
Both Spain and Chile have similar math curricula at the elementary school level. In both Chile ( MINEDUC, 2018 ) and Spain (e.g., Decreto n.° , 198/2014 ), students are doing single-digit addition and subtraction at the end of the first grade (at around age seven). In second grade, they learn multiplication and begin adding and subtracting beyond single digits. In third grade, they begin using multiplication and division to solve math problems. Although both samples are socially and culturally distinct, the objective of this study was to investigate whether gender differences exist and, if so, whether the pattern is the same for the two Spanish-speaking countries. Since both Chile and Spain have similar math curricula, if this was the case, the gender gap could be considered intrinsic.
International evaluations and even math tests administered at schools often do not consider the skills and basic knowledge for acquiring mathematical abilities, and are instead focused on complex calculation or problem solving ( Nosworthy et al., 2013 ). Hence, when gender differences are observed in such tests, it is hard to determine whether such differences are also present for more basic math skills. A logical hypothesis is that the basic abilities needed for complex tasks would also show a gender gap. In recent years, different studies have explored what basic numerical skills necessary for mathematical achievement are responsible for gender differences (for instance, Kersey and Cantlon, 2018 ; Hutchison et al., 2019 ).
In this regard, the literature has widely reported on the basic skills required for mathematical achievement. On one hand, non-symbolic magnitude comparison (e.g., the ability to look at two groups of objects and determine which is greater in number), is considered a stepping stone to learning numbers (see Landerl, 2019 ). For non-symbolic comparison, visuospatial perception, not the ability to count, is required ( Kersey and Cantlon, 2018 ). Once it is acquired, boys and girls can relate quantities with symbolic numbers (for instance, relating the number four with four objects) and learn the meaning of the Arabic numeral. On the other hand, symbolic magnitude comparison (the ability to choose which of two numbers is larger) requires participants to efficiently access the analog magnitude representations that correspond to the Arabic numerals in order to determine which number is greater ( Landerl, 2019 ). Two tasks are widely used to evaluate these abilities: the symbolic magnitude comparison task and the non-symbolic magnitude comparison task. A recent meta-analysis has confirmed that both tasks correlate with subsequent mathematical performance, although the correlation is higher for the symbolic comparison task ( Schneider et al., 2016 ).
Once children understand the meaning of the Arabic numerals, they start to count. The first operations are simple single-digit addition and subtraction with a sum of <10. Depending on one's knowledge of arithmetic facts, two strategies are mainly used. In the beginning, the child will have to count to solve the operation; and through repetition, the answers become lodged in their memory ( De Smedt et al., 2019 ). The automation of these operations has been considered critical to developing robust complex calculations ( Royer et al., 1999 ).
Calculation and mathematical problems are considered more complex areas of mathematical performance. Mathematical problems also require other abilities such as reading comprehension ( Abedi and Lord, 2001 ; Donlan et al., 2007 ). Calculation usually includes the four basic mathematical operations (addition, subtraction, multiplication, and division) and generally cannot be solved from memory, since it usually involves double digits or more. Children can use a wide variety of strategies for approaching these operations, such as decomposition or sequencing ( Hickendorff et al., 2019 ). Calculation also requires executive functions, such as working memory. The strategy children use depends not only on their individual skills but also on the instruction they have received. Gender differences have been found (e.g., Diamantopoulou et al., 2012 ) for these complex tasks. Winkelmann et al. (2008) proposed that disparities in different studies on gender differences can be attributed to what is considered “basic ability” in a particular study and on the cognitive resources required for each task. The breakdown of tasks by gender differences allows for the design of learning and support strategies in specific areas to gradually reduce the gender gap.
Gender differences tend to be more evident in upper elementary. Some studies do present evidence of a gender gap for lower elementary ( Jordan et al., 2006 ; Scheiber et al., 2015 ), but others do not ( Lachance and Mazzocco, 2006 ). Third grade seems to be a critical year for the detection of gender differences in mathematical performance, although the studies at this level are scarce. As an example, Germany presents gender differences starting in third grade ( Winkelmann et al., 2008 ). In Spain, the TIMSS (2015 and 2019) reports a large gender gap in fourth grade, thus suggesting a possible gender gap in third grade as well. Another international study, TERCE 2015 ( UNESCO, 2016 ), shows that the number of Latin American countries where males have an advantage over females increases between third and sixth grade. Therefore, second and third grade are considered crucial. The aim of this study is not to determine the age at which gender differences, if they exist, appear, but to observe whether these differences are detected in lower elementary in Spanish-speaking countries.
This study delves into these differences to determine if they are present in the first years of school for basic math skills. After controlling for reading proficiency, mathematical performance by Spanish-speaking second and third graders in Chile and Spain will be compared for non-symbolic comparison, symbolic comparison, fluency, and calculation. As part of this study, any differences in mean performance, variance in the distribution of performance, and percentiles will be assessed. According to the literature, differences are not expected in basic mathematical tasks such as symbolic and non-symbolic comparison but could appear for tasks that require mathematical fluency and calculation. We hypothesize that a greater number of males will be in the higher percentiles and a greater number of females will be in the lower percentiles.
Methodology
Participants.
The participants were second and third graders at four schools in the O'Higgins region (Chile) and seven schools in Murcia (Spain). These children were organized into two separate samples, one for each country, with a mean age of 7.75 for the second graders ( M = 7.68, SD = 0.31 for the Spanish children, and M = 7.88, SD = 0.48 for the Chilean children) and 8.77 for the third graders ( M = 8.7, SD = 0.33 for the Spanish children, and M = 8.89, SD = 0.39 for the Chilean children). The sample was formed by 201 Spanish school children (107 second graders and 94 third graders; 55.2% male and 44.8% female) and 184 Chilean school children (90 second graders and 94 third graders; 48.9% male and 51.1% female) and was collected through incidental sampling. The following exclusion criteria were established: vision difficulties, diagnosis of autism spectrum disorder or cognitive impairment, insufficient knowledge of the Spanish language, and, in the case of the third graders, significant difficulties reading. Table 1 details the number of males and females by grade and country. Possible differences regarding age (Mann–Whitney U) and gender proportion by grade and country (χ 2 ) were examined.
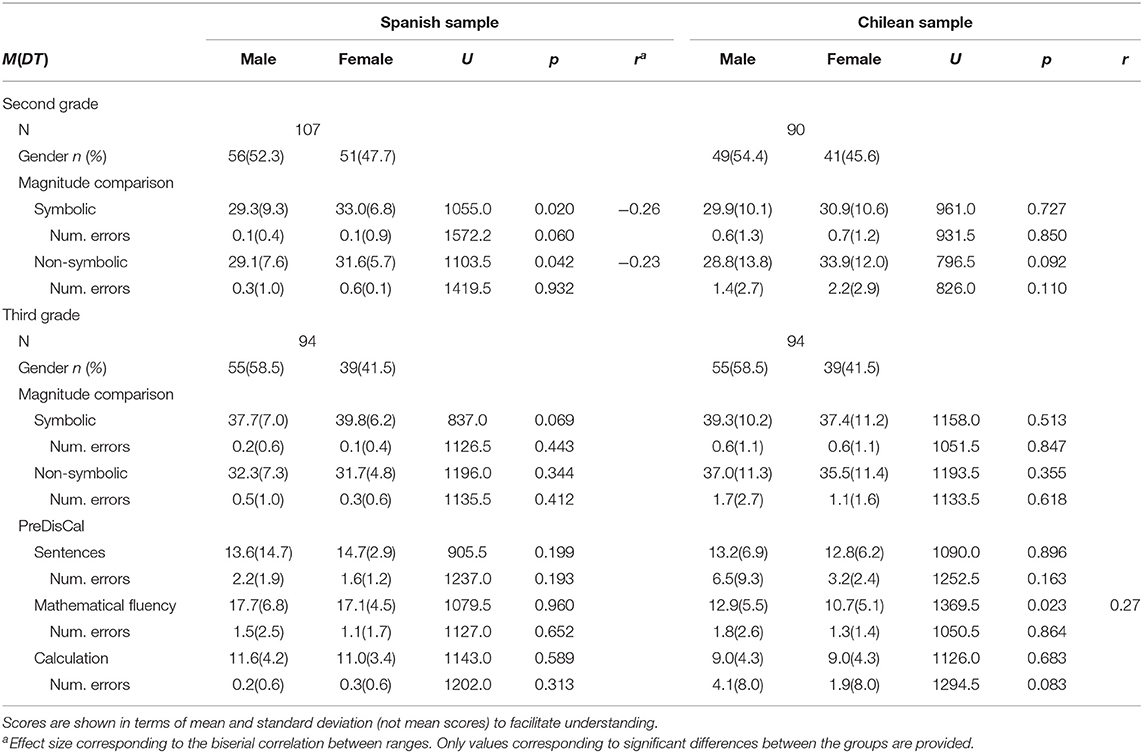
Table 1 . Distribution in the Spanish language sample of second and third graders: scoring on symbolic test by gender.
This study was carried out following the recommendations of the Chilean Commission for Scientific and Technological Investigation (CONICYT in its Spanish acronym). The protocol was approved by the Ethics Committee of Universidad de la Frontera (Act 066-2017, on Sheet 036-17). The study complied with the standards of the ethical committee of Universidad Autónoma de Chile and with the agreement between the Department of Education and Universities of the autonomous community of Region of Murcia and Universidad de Murcia. Informed consent was requested to participate in this study.
Instruments
The following paper-and-pencil tests were administered to measure mathematical performance and reading fluency.
Numerical magnitude comparisons ( Nosworthy et al., 2013 ). This task includes two different parts:
- Symbolic task (56-digit pairs), where the participants were asked to compare numerical pairs (numbers one through nine) and indicate which number was higher.
- Non-symbolic task (56 dot arrays), where dot arrays of varying quantities are present and the participants were asked to indicate which side had more dots.
Each test had a time limit of 1 min, and the dependent variable was the number of correct answers.
PreDisCal ( Pina et al., 2020 ). This is a set of three tests that measure reading fluency, mathematical fluency, and calculation, in that order. Although the PreDisCal scale was developed in Spain, a cross-validation process was carried out in several Latin American countries. Some of the items were modified because of interpretation issues (related to sociocultural factors) in Latin American countries. The test included the following tasks:
- Sentences. This task consists of 47 sentences that assess reading fluency. Each sentence has a missing word, for instance: “The strawberries are …” followed by five answer options (one is correct). The incorrect alternatives are close grammatically or semantically. All the sentences are easy to understand. The test had a time limit of 3 min. The dependent variable is the number of correct answers. It is a validated test with adequate test-retest reliability [ r (169) = 0.8, p < 0.001].
- Mathematical fluency. The arithmetic facts are tested through 63 single-digit addition and subtraction operations. All sums are < 10. The maximum time is 1 min in this test, and the dependent variable is the number of correct answers. The reliability of the test is adequate [ r (169) = 0.85, p < 0.001].
- Calculation. This test comprises 45 items of increasing difficulty and assesses complex calculation. Participants have to determine what number or symbol, or if both are missing in a comparison of two operations (e.g., “3 + _ = 5 + 1”). The test had a time limit of 3 min, and the dependent variable was the number of correct answers. The test-retest reliability of the validated scale was acceptable [ r (169) = 0.75, p < 0.001].
The tasks were administered in February for Spain and in September of the same year for Chile in order to reflect the same moment of the academic calendar in both countries. Trained evaluators collectively administered the test during the school day. Given that PreDisCal required a certain degree of reading proficiency and complex calculation ability, it was administered only to the third graders, while the magnitudes comparison tests were administered to both the second and third grade samples. In the case of the magnitude comparisons, the symbolic test was administered first, followed by the non-symbolic test. The PreDisCal was administered in keeping with the order established for that test, e.g., sentences, mathematical fluency, and calculation.
Data Analysis
First, the comparability of the groups was verified by assessing age and gender differences by grade and country (χ 2 ), and mean performance in the sentences test between countries (Student t). Second, basic parametric assumptions for data analysis were verified (normality using the Kolmogorov–Smirnov test, homoscedasticity using the Levene test). Non-parametric tests were carried out using the Mann–Whitney U and statistical biserial correlations ( r = 0.1 as low; r = 0.3 as medium, and r = 0.5 as high effect; Cohen, 1988 ); and χ 2 to compare gender and percentile performance distribution in third grade.
Both samples showed a similar gender distribution of participants (χ 2 = 0.09, p = 0.768 for second grade; χ 2 = 0, p = 1 for second grade). There was no significant evidence between countries in relation to the mean performance in the sentences test ( t = 1.35; p = 0.178). However, significant differences were detected between countries regarding the mean age of students ( t = −5.04, p < 0.001, d = −0.52 for the third grade; t = −4.18, p < 0.001, d = −0.86 for the second grade), with moderate to high effect sizes ( Cohen, 1988 ). Nevertheless, these differences in age are not relevant to this study, since the analysis between countries is carried out comparing gender, a variable for which there are no significant differences in the samples of both countries.
The distribution of data was examined for each dependent variable according to country and course. Except for the mathematical fluency and calculation tests in the Chilean third grade sample, the remaining variables followed a non-normal distribution (K-S < 0.05); thus, non-parametric analyses were applied.
The Levene test showed significant difference in variance between genders in the symbolic test for the Spanish sample of second graders ( F = 3.97, p = 0.049). The scores of boys showed higher variability (σ 2 = 86.5) with skewness of 0.18, ( SE = 0.32) and kurtosis of −0.19 ( SE = 0.63), compared with those of the girls (σ 2 = 46.3) with skewness of 0.1 ( SE = 0.33), and kurtosis of 0.27 ( SE = 0.66), as shown in Figure 1 . Differences in variance were not found for the other tests for either sample.
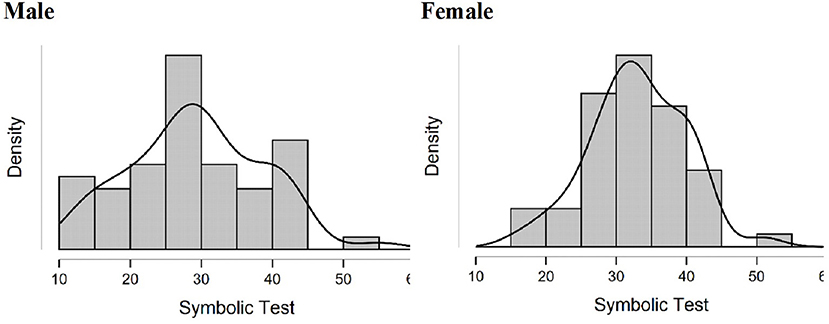
Figure 1 . Distribution in the Spanish language sample of third grader scores on the symbolic test by gender.
Spearman's correlation was carried out between tests for each country ( Table 2 ). The correlation between scores on the symbolic and non-symbolic tests was crucially positive and high in the second grade sample. As for the third graders, mathematical fluency positively correlated to calculation and with sentences; these last two subscales correlated positively. The symbolic test also correlated positively with the non-symbolic one ( r s = 0.46, p < 0.001).
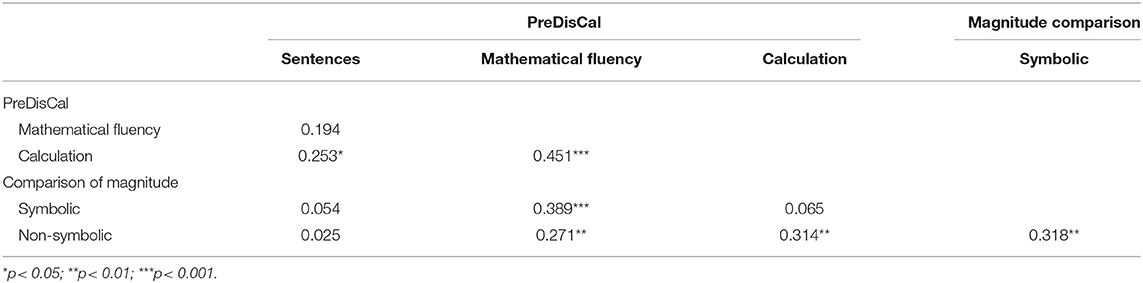
Table 2 . Spearman's correlations between the PreDisCal and magnitude comparison (third grade tests).
Mean Performance by Gender and Grade
If differentiated by gender, the Mann–Whitney tests indicated that the girls scored significantly higher (See Table 2 ) in the symbolic test of the Spanish second graders ( U = 1,055, p = 0.02, r = −0.26), but this was not the case for the Chilean sample ( p = 0.727). However, the effect size of the significant differences ranged from low to moderate. Regarding the non-symbolic test, the results were similar, with girls outperforming the boys in the Spanish sample ( U = 1,103, p = 0.42, r = −0.23), but not in the Chilean sample ( p = 0.092). In this case, the effect size was lower than in the symbolic test. The differences detected in the sample disappear in the third grade. In terms of the number of mistakes made on both tests, no significant differences were found between genders in either country.
In the third-grade sample, significant differences were only found between genders for the mathematical fluency test in the Chilean sample ( U = 1,369, p = 0.023, r = 0.27). Here, the girls had lower scores ( Md = 9) than the boys ( Md = 13). In terms of the number of mistakes made on both tests, no significant differences were found between genders in either country at the third grade level.
Ratio of Boys to Girls at Various Percentiles
Four groups for each sample were formed according to the scores they obtained and their corresponding percentiles. Group 1 scored in the 1–25th percentile; group 2 in the 26–50th percentile; group 3 in the 51–75th percentile; and group 4 in the 76th percentile or higher. χ 2 and its significance were calculated to examine significant differences in the ratio of males and females in the different percentile groups. As no significant differences were found in terms of performance of boys and girls in the third-grade tests (except for mathematical fluency, which had a low effect size), the groups were formed by students from both countries. In the case of the second graders, the calculations varied by country.
For the third group, there were no significant gender differences in terms of the ratio of boys to girls, the different percentile groups, or for any of the tests (see Table 3 ). However, significant differences were found in the symbolic test for the Spanish sample of second graders (χ 2 = 9.65, p = 0.022). The percentages were different for the first group (scorings between the 1–25th percentile; χ 2 = 6.54, p = 0.011), with a larger quantity of boys (30.4%) than girls (9.8%). Significant differences were not found in the Chilean sample of second graders.
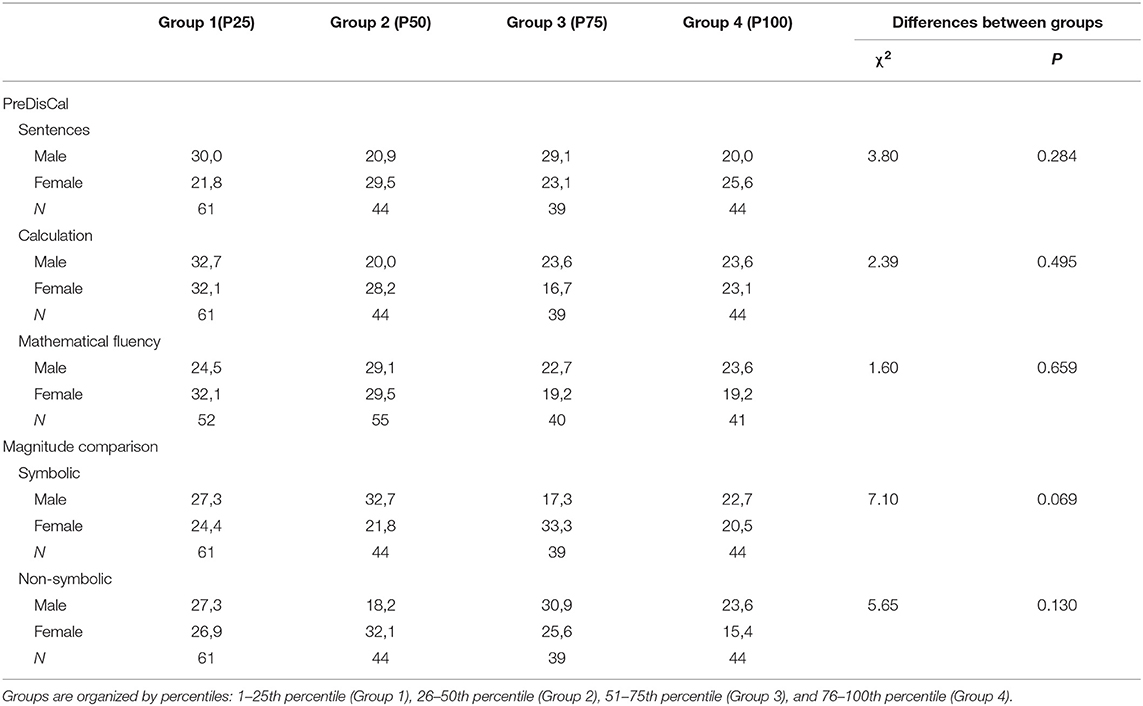
Table 3 . Percentage and comparison of third grade boys and girls by groups corresponding to the 25th, 50th, 75th, and 100th percentile.
This study set out to compare the basic mathematical abilities of boys and girls, which prove essential for the acquisition of complex math skills. The sample was composed of boys and girls from two Spanish-speaking yet culturally different countries in the second and third grade, ages at which the gender gap starts to appear. The results allow us to conclude, in keeping with the scientific literature on the topic, that in terms of basic mathematical knowledge, boys and girls show no significant differences in mathematical performance. Previous investigations, including various meta-analyses ( Lachance and Mazzocco, 2006 ; Hyde et al., 2008 ; Lindberg et al., 2010 ; Voyer and Voyer, 2014 ; Scheiber et al., 2015 ), have noted as much. Similarly, Hutchison et al. (2019) found no differences in numerical and magnitude comparison, numerical ordering, magnitude estimation, multiplication, and division, although the population analyzed for their study hailed from the Netherlands, meaning that results could be different in countries with a higher gender gap. The results of this study, however, reveal no significant differences in most of the tasks considered for two Spanish-speaking samples from Chile and Spain, despite the high gender gap detected in international tests for the two countries (such as TIMSS 2019).
The main differences are found in the symbolic test (comparison of two numbers to determine which one is higher) in the Spanish sample of second graders. In particular, the girls performed better and the boys showed a greater variability. Additionally, females had a higher mean performance, and a greater ratio of males fell below the 25th percentile. However, the effect size is small in second grade, and no such results were found in the third grade sample. For the non-symbolic comparison test, the girls performed better once again, although this difference disappeared in third grade. The direction of these gender differences goes against the hypothesis of higher performance in males. In line with these results, previous studies have shown gender differences in favor of females in basic abilities (for instance, Halpern and Wright, 1996 ).
The only difference in favor of males was found in the Chilean sample in average performance for mathematical fluency in the third grade. Differences in addition and subtraction have been found previously, although there has not always been evidence of these differences ( Hutchison et al., 2019 ). In this case, the effect size was small and no differences were found for calculation, meaning that caution is critical when interpreting the results. In the case of the Chilean third graders, test abilities were recently acquired, and the differences would likely disappear with practice. Importantly, mathematical fluency is decisive for the robust development of complex calculation and is an ability that can be practiced until it is learned ( Royer et al., 1999 ).
Prior studies have indicated that differences in mathematical performance rely on the kind of task students are asked to complete. These studies have found that females perform better in arithmetic and calculus, while males perform better in mathematical problem solving ( Byrnes and Takahira, 1993 ). However, there have also been conflicting results in the research. For instance, Royer et al. (1999) identified that males perform better in math-fact retrieval than females. In another study, Winkelmann et al. (2008) showed that females had poorer basic skills, while males had a slight advantage in mathematical problems, such as equations containing a missing number. The authors explained that the results depended on basic elementary skills and on how math problems are defined, i.e., on the cognitive resource that each task demanded.
One hypothesis is that gender differences can be found in complex areas of mathematics that demand more cognitive resources. In this sense, for example, the literature suggests a relationship between spatial abilities and gender differences in mathematical performance. However, a recent study did not confirm these results and instead concluded that gender differences may depend on the test and the strategy used to solve each item ( Ramírez-Uclés and Ramírez-Uclés, 2020 ). The results support this hypothesis. In particular, the calculation is the task that involves the highest cognitive demand, but no gender differences were found. Another possibility is that gender differences in mathematical performance emerge later in more complex mathematical tasks or are influenced by cultural or social stereotypes, although the results show that at least lower elementary school children do not exhibit such differences.
Another hypothesis for gender differences in mathematical performance is related to the way in which the answer options are presented on tests. For instance, in a study with PISA 2000 data, Lafontaine and Monseur (2009) found that in all the countries analyzed, open-ended (as opposed to multiple choice) answers for reading tasks favored females. For mathematics, Routitsky and Turner supported these conclusions using PISA 2003 data but pointed out that this difference disappeared as the complexity of items increased ( Routitsky and Turner, 2003 ). Future investigations should assess how the questions and type of answer options influence the gender gap.
Previous studies have revealed that the learning routine shows no differences between genders, although boys and girls do express different attitudes toward mathematics ( Barbero-García et al., 2007 ). This could explain why differences can be found in higher grades despite being absent when basic math skills are evaluated. Importantly, gender differences may well be related to the wording of tasks or instructions, or social behaviors that influence how parents, teachers, and even female students view their own mathematical ability, not by any intrinsic math difficulties (see Lindberg et al., 2010 ).
This study presents several limitations. All the tasks considered had a time limit, which can influence the results. Processing speed is essential for school performance ( Dodonova and Dodonov, 2012 ), and it is important for the diagnosis of learning disabilities, since children with a diagnosis are identical to those with mean performance if we provide them sufficient time ( Jordan and Montani, 1997 ). The second constraint of this study is that no information was obtained on the socioeconomic level of the children. However, both samples attended public schools, and none were disadvantaged. Lastly, this study is exploratory, and despite having a large sample, it cannot be deemed representative of the total population of both countries.
Gender differences in mathematical performance have been less studied in Spanish-speaking countries. This study was laid out as an initial approach to researching this population. Based on existing literature and the results, gender differences vary by task, strategies used to solve problems, cohort, age, and instructions provided. For future research, the sample should be expanded geographically for higher representativeness in relation to social, educational, and cultural differences. Furthermore, it would be interesting to expand the number of courses evaluated to observe the pattern between genders and to include more complex tasks; this should allow specific mathematical areas to be identified in which gender differences can easily be detected ( Chacón-Moscoso et al., 2019 ). These new developments would potentially reveal differences related to the country, factorial invariance, differential item functioning (DIF), and the influence of open-ended (as opposed to multiple choice) questions ( Chacón-Moscoso et al., 2016 ).
Practical Value, Implications for Educational Intervention
It should be noted that the tests applied in this study could be used for an early diagnosis of gender difference, and with respect to children with low mathematical performance.
Conclusions
In conclusion, results emphasizing that males outperform females in math do not hold true in the key mathematical areas analyzed with the exception of mathematical fluency in the Chilean sample, where the effect size was small. Therefore, the acquisition of complex abilities should be the same for males and females. At a scientific level and in the news media, care should be exercised when reporting on gender differences because it can influence own opinion of the girls of their mathematical ability. To the best knowledge of the authors, this study is the first to examine gender differences in basic math skills in Spanish-speaking populations that reveals considerable gaps in international studies on the topic.
Data Availability Statement
The raw data supporting the conclusions of this article will be made available by the authors, without undue reservation.
Ethics Statement
The studies involving human participants were reviewed and approved by Chilean Commission for Scientific and Technological Investigation (CONICYT, in its Spanish acronym). The protocol was approved by the Ethics Committee of Universidad de la Frontera (Act 066-2017, on Sheet 036-17). The study was in accordance with the standards of the ethical committee of Universidad Autónoma de Chile and with the agreement between the Region of Murcia's Comunidad Autónoma through the Department of Education and Universities, and Universidad de Murcia. Written informed consent to participate in this study was provided by the participants' legal guardian/next of kin.
Author Contributions
VP, JF-C, and DM conceptualized and designed the study. MS acquired the data. VP, JF-C, and SC-M analyzed and interpreted the data. VP, JF-C, and DM wrote the manuscript. JF-C prepared the figures and tables. SC-M discussed results and reviewed writing. All the authors read and approved the final version of the manuscript.
This study has been supported by the Chilean national projects Fondecyt Regular 2018 ID1181472, Anid/Fondecyt Regular ID1210172 and Fondecyt Regular 2019 (ref. number 1190945) and by a fellowship for residencies abroad of young researchers from Mare Nostrum (2017). Also, this research has been supported by the ERDF Andalusia Operational Program 2014–2020, government of Andalusia, Spain (ref. US-1263096); the VI Own Research and Transfer Plan (VIPPITUS), Universidad de Sevilla, Spain (ref. VIPP PRECOMPETI 2020/1333).
Conflict of Interest
The authors declare that the research was conducted in the absence of any commercial or financial relationships that could be construed as a potential conflict of interest.
Publisher's Note
All claims expressed in this article are solely those of the authors and do not necessarily represent those of their affiliated organizations, or those of the publisher, the editors and the reviewers. Any product that may be evaluated in this article, or claim that may be made by its manufacturer, is not guaranteed or endorsed by the publisher.
Abedi, J., and Lord, C. (2001). The language factor in mathematics tests. Appl. Measure. Edu. 14, 219–234. doi: 10.1207/S15324818AME1403_2
CrossRef Full Text | Google Scholar
Adams, R. B., Barber, B. M., and andOdean, T. (2019). The Math Gender Gap and Women's Career Outcomes. Available online at SSRN: https://ssrn.com/abstract=2933241 (accessed December 17, 2019).
Google Scholar
Anaya, L., Stafford, F. P., and andZamarro, G. (2017). Gender Gaps in Math Performance, Perceived Mathematical Ability and College STEM Education: The Role of Parental Occupation. EDRE Working Paper No. 2017-21. Available online at SSRN: https://ssrn.com/abstract=3068971 (accessed November 10, 2017).
Baker, D. P., and Jones, D. P. (1993). Creating gender equality: cross-national gender stratification and mathematical performance. Sociol. Edu. 66, 91–103. doi: 10.2307/2112795
Barbaresi, W. J., Katusic, S. K., Colligan, R. C., Weaver, A. L., and Jacobs, S. J. (2005). Math learning disorder: incidence in a population-based birth cohort, 1976 – 82, Rochester, Minn. Ambul. Pediatr. 5, 281–289B. doi: 10.1367/A04-209R.1
PubMed Abstract | CrossRef Full Text | Google Scholar
Barbero-García, I., Holgado-Tello, F. P., Vila-Abad, E., and Chacón-Moscoso, S. (2007). Actitudes, hábitos de estudio y rendimientoenMatemáticas: diferencias por género. Psicothema 19, 413-421. Available online at: http://www.psicothema.com/pdf/3379.pdf
Belloum, A. S. Z., Koulouzis, S., Wiktorski, T., and andManieri, A. (2019). Brinding the demand and the offer in data science. Concurrency Comput. Pract. Exp. 31:E5200. doi: 10.1002/cpe.5200
Byrnes, J. P., and Takahira, S. (1993). Explaining gender differences on SAT-math items. Dev. Psychol . 29, 805–812. doi: 10.1037/0012-1649.29.5.805
Chacón-Moscoso, S., Anguera, M. T, Sanduvete-Chaves, S., Losada-López, J. L., Lozano-Lozano, J. A., and Portell, M. (2019). Methodological quality checklist for studies based on observational methodology (MQCOM). Psicothema 31, 458–464. doi: 10.7334/psicothema2019.116
Chacón-Moscoso, S., Sanduvete-Chaves, S., and Sánchez-Martín, M. (2016). The development of a checklist to enhance methodological quality in intervention programs. Front. Psychol. 7:1811. doi: 10.3389/fpsyg.2016.01811
Cohen, J. (1988). Statistical Power Analysis for the Behavioral Sciences , 2nd Edn. Lawrence Erlbaum Associates.
PubMed Abstract | Google Scholar
De Smedt, B., Peters, L., and Ghesquière, P. (2019). “Neurobiological origins of mathematical learning disabilities or dyscalculia: a review of brain imaging data,” in International Handbook of Mathematical Learning Difficulties , eds A.Fritz, V. G. Haase, & P. Räsänen (Cham, Switzerland: Springer), 367–384. doi: 10.1007/978-3-319-97148-3_23
Decreto n.° (198/2014). BORM de 5 de septiembrepor el que se establece el currículo de la Educación Primaria en la Comunidad Autónoma de la Región de Murcia (Región de Murcia: Boletin Oficial de la Region de Murcia (BORM), n° 206, 2014).
Diamantopoulou, S., Pina, V., Valero-Garcia, A., González-Salinas, C., and Fuentes, L. J. (2012). Validation of the Spanish version of the woodcock–johnson mathematics achievement tests for children aged 6 to 13. J. Psychoedu. Assess. 30, 466–477. doi: 10.1177/0734282912437531
Dodonova, Y. A., and Dodonov, Y. S. (2012). Processing speed and intelligence as predictors of school achievement: Mediation or unique contribution? Intelligence 40, 163–171. doi: 10.1016/j.intell.2012.01.003
Donlan, C., Cowan, R., Newton, E. J., and Lloyd, D. (2007). The role of language in mathematical development: Evidence from children with specific language impairment. Cognition 103, 23–33. doi: 10.1016/j.cognition.2006.02.007
Halpern, D. F., and Wright, T. M. (1996). A process-oriented model of cognitive sex differences. Learn. Indiv. Dif. 8, 3–24. doi: 10.1016/S1041-6080(96)90003-5
Havelock Ellis (1894). Man and Woman: A Study of Human Secondary Sexual Characters . New york, NY: Scribner's.
Hickendorff, M., Torbeyns, J., and Verschaffel, L. (2019). “Multi-digit addition, subtraction, multiplication, and division strategies,” in International Handbook of Mathematical Learning Difficulties , eds A. Fritz, V. Haase& P. Räsänen (Cham, Switzerland: Springer), 543–560. doi: 10.1007/978-3-319-97148-3_32
Hutchison, J., Lyons, I., and Ansari, D. (2019). More similar than different: gender differences in basic numeracy are the exception, not the rule. Child Dev. 90, 66000–79000. doi: 10.1111/cdev.13044
Hyde, J. S., Lindberg, S. M., Linn, M. C., Ellis, A. B., and Williams, C. C. (2008). Gender similarities characterize math performance. Science 321, 494–495. doi: 10.1126/science.1160364
Instituto Nacional de Evaluación Educativa [INEE] (2016). TIMSS 2015. Estudio internacional de tendencias en Ciencias y Matemáticas. IEA. Informe español: Resultados y contexto. Madrid : Ministerio de Educación, Cultura y Deporte.
Instituto Nacional de Evaluación Educativa [INEE] (2020). TIMSS 2019. Estudio internacional de tendencias en Ciencias y Matemáticas. IEA. Informe español: Resultados y contexto. Madrid : Ministerio de Educación, Cultura y Deporte.
Jordan, N. C., Kaplan, D., Olah, L., and Locuniak, M. N. (2006). Number sense growth in kindergarten: a longitudinal investigation of children at risk for mathematics difficulties. Child Dev. 77, 153–175. doi: 10.1111/j.1467-8624.2006.00862.x
Jordan, N. C., and Montani, T. O. (1997). Cognitive arithmetic and problem solving: a comparison of children with specific and general mathematics difficulties. J. Learn. Disabil. 30, 624–634. doi: 10.1177/002221949703000606
Kane, J. M., and Mertz, J. E. (2012). Debunking myths about gender and mathematics performance. Not. AMS 59, 10–21. doi: 10.1090/noti790
Kersey, A. J.;, Braham, E. J.;, Csumitta, K. D.;, Libertus, M. E., and Cantlon, J. F. (2018). No intrinsic gender differences in children's earliest numerical abilities. NPJ Sci. Learn. 3, 1–10. doi: 10.1038/s41539-018-0028-7
Lachance, J. A., and Mazzocco, M. M. (2006). A longitudinal analysis of sex differences in math and spatial skills in primary school age children. Learn. Indiv. Dif. 16, 195–216. doi: 10.1016/j.lindif.2005.12.001
Lafontaine, D., and Monseur, C. (2009). Gender gap in comparative studies of reading comprehension: to what extent do the test characteristics make a difference? Eur. Edu. Res. J. 8, 69–79. doi: 10.2304/eerj.2009.8.1.69
Landerl, K. (2019). “Neurocognitive Perspective on Numerical Development,” in International Handbook of Mathematical Learning Difficulties , eds A. Fritz, V. G. Haase, and P. Räsänen (Cham, Switzerland: Springer), 9–25. doi: 10.1007/978-3-319-97148-3_2
Lindberg, S. M., Hyde, J. S., Petersen, J. L., and Linn, M. C. (2010). New trends in gender and mathematics performance: a meta-analysis. Psychol. Bull. 136, 1123–1135. doi: 10.1037/a0021276
MINEDUC (2018). Bases Curriculares Primero a Sexto Básico . Santiago: Ministerio de Educacion.
Nosworthy, N., Bugden, S., Archibald, L. A., Evans, B., and andAnsari, D. (2013). A two-minute paper and pencil test of symbolic and nonsymbolic numerical magnitude processing explains variability in primary school children's arithmetic competence. PLoS ONE 8:e67918. doi: 10.1371/journal.pone.0067918
Pina, V., Hernández-Pérez, E., Rabadán-Rubio, J. A., Hernández-Pallarés, L., and andFenollar-Cortés, J. (2020). PreDisCal: Prueba de cribado para la detección de dificultades lectoras o matemáticas de segundo a sexto de primaria . Madrid: TEA Ediciones.
Ramírez-Uclés, I. M., and Ramírez-Uclés, R. (2020). Gender differences in visuospatial abilities and complex mathematical problem solving. Front. Psychol. 11:191. doi: 10.3389/fpsyg.2020.00191
Reigosa-Crespo, V., Valdes-Sosa, M., Butterworth, B., Estevez, N., Rodriguez, M., Santos, E., et al. (2012). Basic numerical capacities and prevalence of developmental dyscalculia: the havana survey. Dev. Psychol. 48, 123–135. doi: 10.1037/a0025356
Routitsky, A., and Turner, R. (2003). “Item format types and their influences on cross-national comparisons of student performance,” in Paper Presented at the Annual Meeting of the American Educational Research Association (Chicago).
Royer, J. M., Tronsky, L. N., Chan, Y., Jackson, S. G., and Marchant, H. G. (1999). Math fact retrieval as the cognitive mechanism underlying gender differences in math achievement test performance. Contemp. Edu. Psychol. 24, 181–266. doi: 10.1006/ceps.1999.1004
Scheiber, C, Reynolds, M. R., Hajovsky, D. B., and Kaufman, A.S. (2015). Gender differences in achievement in a large, nationally representative sample of children and adolescents. Psychol. Schools 52, 335–348. doi: 10.1002/pits.21827
Schneider, M., Beeres, K., Coban, L., Merz, S., Schmidt, S. S., Stricker, J., and DeSmedt (2016). Association of non-symbolic and symbolic numerical magnitude processing with mathematical competence: a meta-analysis. Dev. Sci. 20:e12372. doi: 10.1111/desc.12372
Shields, S. A. (1982). The variability hypothesis: the history of a biological model of sex differences in intelligence. Signs. J. Women Cult. Soc. 7, 769–797. doi: 10.1086/493921
UNESCO, Gelber. (2016). Inequidad de género en los logros de aprendizaje en educación primaria? ‘Qué nos puede decir TERCE? Santiago: Laboratorio Latinoamericano de Evaluación de la Calidad de la Educación.
Voyer, D., and Voyer, S. D. (2014). Gender differences in scholastic achievement: a meta-analysis. Psychol. Bull. 140, 1174–1204. doi: 10.1037/a0036620
Winkelmann, H., van den Heuvel-Panhuizen, M., and Robitzsch, A. (2008). Gender differences in the mathematics achievements of German primary school students: results from a German large-scale study. ZDM 40, 601–616. doi: 10.1007/s11858-008-0124-x
Keywords: gender differences, primary education, mathematical fluency, calculation, children
Citation: Pina V, Martella D, Chacón-Moscoso S, Saracostti M and Fenollar-Cortés J (2021) Gender-Based Performance in Mathematical Facts and Calculations in Two Elementary School Samples From Chile and Spain: An Exploratory Study. Front. Psychol. 12:703580. doi: 10.3389/fpsyg.2021.703580
Received: 30 April 2021; Accepted: 08 July 2021; Published: 17 August 2021.
Reviewed by:
Copyright © 2021 Pina, Martella, Chacón-Moscoso, Saracostti and Fenollar-Cortés. This is an open-access article distributed under the terms of the Creative Commons Attribution License (CC BY) . The use, distribution or reproduction in other forums is permitted, provided the original author(s) and the copyright owner(s) are credited and that the original publication in this journal is cited, in accordance with accepted academic practice. No use, distribution or reproduction is permitted which does not comply with these terms.
*Correspondence: Violeta Pina, violetapina@ugr.es ; Diana Martella, diana.martella@uautonoma.cl
Disclaimer: All claims expressed in this article are solely those of the authors and do not necessarily represent those of their affiliated organizations, or those of the publisher, the editors and the reviewers. Any product that may be evaluated in this article or claim that may be made by its manufacturer is not guaranteed or endorsed by the publisher.
- Subscribe Newsletter
- Track Paper
- Conferences

International Journal of Research and Innovation in Social Science (IJRISS)
- ISSN No. 2454-6186
- Strengthening Social Sciences for the Future
- May Issue 2024
- Research Area
- Initial Submission
- Revised Manuscript Submission
- Final Submission
- Review Process
- Paper Format
- Author (s) Declaration
- Registration
- Virtual Library
- Apply as Reviewer
- Join as a Board Member
- Eligibility Details & Benefits
- Board Members
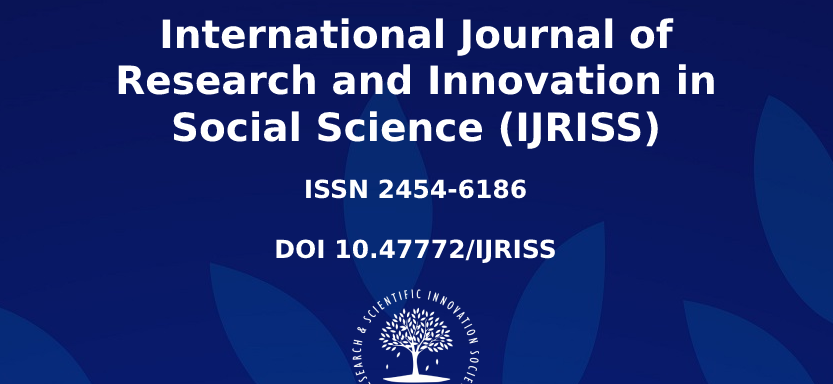
Strategies for Inculcating Gender Equity in Mathematics Classrooms for Enhancement of Learners’ Problem-Solving Skills.
- Dennis R. Nimely, Jr.
- Florence K. Nyamu, OGW (PhD)
- Aug 15, 2023
- Social Science
1 Dennis R. Nimely, Jr.*, 2 Florence K. Nyamu, OGW (PhD)
1 Post-graduate Candidate, Department of Educational Communication and Technology, School of Education, Kenyatta University
2 Senior Lecturer, Department of Educational Communication and Technology, School of Education, Kenyatta University
* Corresponding Author
DOI: https://dx.doi.org/10.47772/IJRISS.2023.70814
Received: 30 June 2023; Revised: 17 July 2023; Accepted: 20 July 2023; Published: 15 August 2023
The report released in 2022 by United Nations Educational, Scientific, and Cultural Organization (UNESCO) shows that globally, female enrolment is at least 88% at primary and secondary school levels. The 88% is an indicator of good progress in gender equality in education. Of concern, however, is gender equity in Mathematics classrooms. Internationally set goals, Education For All (EFA) Goal 5, Millenium Development Goals (MDGs) Goal 3 and Sustainable Development Goals (SDGs) Goal 4, all focus on gender equity. This study aims to raise awareness about strategies and practices for gender equity in teaching and learning Mathematics. The study uses inclusion and exclusion criteria, a literature review, and empirical data analysis. Evidence suggests that gender equity in Mathematics improves critical thinking skills and increases gender balance in Mathematics education. The researchers recommend further study on strategies for integrating gender equity in Mathematics classrooms.
Keywords: Gender equity, problem-solving, collaborative learning
INTRODUCTION
The effort of global institutions to combat social injustice and gender inequity and inequality is gaining momentum, with a Global Education Monitoring (UNESCO, 2022) report reporting at least 88% female enrolment. The progress reports of Education for All (EFA) (UNESCO, 2015) and the Millennium Development Goals (MDGs) show that out of the world’s total population of all school-going children, at least 57 million are still out of school. Also, the issue of gender equity is still a major challenge in many nations.
The Sustainable Development Goals (SDGs), which were introduced to supplement the previous goals, also meant huge challenges, especially during the COVID-19 crisis. Addressing these concerns, global educational institutions have written plans to improve and strengthen gender balance in education. In 1990, UNESCO launched a ten-year plan with six (6) goals, for attainment Education for All (EFA) by the year 2000. The six (6) goals were: i) early childhood care and education; ii) universal primary education; iii) youth and adult skills; iv) adult literacy; v) gender parity and equality; and vi) quality of education.
In EFA goals 5 and 6, they stressed the importance of gender equality and quality education. The world further expands the quest for improvement in all sectors of life by developing two subsequent plans, the Millennium Development Goals (MDGs) and the Sustainable Development Goals (SDGs). There were eight (8) goals of the MDGs: i) eradicate extreme poverty and hunger, ii) achieve universal primary education, iii) promote gender equity and empower women, iv) and v) reduce child mortality and improve maternal health, vi) combat HIV/AIDs, malaria and other diseases, vii) ensure environmental sustainability, and viii) develop a global partnership for development. MDGs 3 emphasises the promotion of gender equality. The most recent global goals of development are the Sustainable Development Goals (SDGs). There are 17 goals, with goals 3, 4, 5, and 16 stressing the need for good health and wellbeing, quality education, gender equality, peace, justice, and strong institutions in the world.
The Kenya Vision 2030, under the social pillar, the government of Kenya pays special attention to gender sensitivity intervention and voucher systems in response to global concerns. It has recently come to the attention of international community that gender equity is a defining characteristic of an inclusive society that recognises and appreciates the contributions made by all its members. It is also an important factor in the consolidation of democracy. As a result, gender equity has emerged as one of the most prominent issues in the ongoing efforts for reform in education around the world.
According to Mawarti and Nurlaelah (2020), Mathematics education is one of the tools for achieving the Sustainable Development Goals (SDGs). They argue that developing strong problem-solving skills in learners through Mathematics education can or will easily speed up the process of achieving the SDGs. The researchers added that integrating and implementing social justice and gender equity in Mathematics classrooms is one of the surest ways of achieving all seventeen goals of the SDGs. It is important that, in order to develop a strong analytical and critical thinking skills, build effective problem-solving skills in learners, and also improve society, gender equity be taken seriously in all aspects of education, especially in Mathematics classrooms (Vale et al., 2016; Vale et al., 2020).
Mathematics educators and curriculum developers consider social justice and gender equity in Mathematics classrooms as an important component in teaching and learning of Mathematics. Problem-solving, which is at the heart of teaching and learning of Mathematics is not only a process skill, but also as a teaching strategy. Spielman (2008), Ty (2011), Wang and Degol (2016), Morris and Jacobi (2022), have described instances of social injustice and gender inequities and how these have impeded the ability of learners in problem-solving in Mathematics classrooms. The findings of Akala and Divala (2016) and Akala (2019), show that some group of individuals believe that men and women are fundamentally different, and as such, society should treat people based on their gender, especially in institutional settings. These beliefs and perceptions have, over the years, promoted social injustice and gender inequities and inequalities (McCray, 2022; Morris & Jacobi, 2022). Despite efforts to increase enrolment and attainment for female learners in education, social injustice and gender inequity remain a major impediment for female learners in Mathematics education.
Researchers have identified social justice and gender equity as two critical dimensions for improving learners’ problem-solving skills in Mathematics. These constructs, according to Ergasheva (2021), are crucial because they improve learners’ attitudes towards learning, encourage more learners of diverse socio-economic status to take part in Mathematics and help students develop their capacity for critical thinking and problem-solving. This paper focuses on gender equity in teaching and learning Mathematics and its influence on learners’ problem-solving skills. It is based on a review of literature that focuses on gender equity in teaching and learning Mathematics. The objective of the study is to identify strategies for integrating gender equity in teaching and learning Mathematics.
LITERATURE REVIEW
Gender equity in teaching and learning Mathematics
According to Rodriguez Martnez et al. (2020) and Rodriguez and Suriel (2022), gender inequities have impeded the progress of Mathematics education, particularly in problem-solving, making Mathematics appear more favourable for male learners. As a result of this, all Mathematics educators and researchers should get involved in creating awareness of the importance of gender equity in Mathematics classrooms, as well as ensuring that both male and female learners perform well in Mathematics at all levels of education. Gender equity as a global concern was incorporated by the Organisation for Economic Co-operation and Development (OECD) in reviewing and integrating equity into their curriculum (For, 2021).
Gender equity refers to the idea that all people, regardless of gender, should have equal opportunities, treatment, and outcomes. Rodriguez and Suriel (2022) described equity as involving and creating a level playing field where individuals of all genders have equal access to resources, opportunities, and rights and where any gender-based barriers or discrimination are addressed and eliminated. In education, specifically in Mathematics education, it implies creating an equitable learning environment that is inclusive, supportive, and motivating for all learners, regardless of gender (Andreasson et al., 2015; Idin & Donmez, 2017).
An equitable learning environment in a Mathematics classroom implies a situation in which learners are given equal opportunities to succeed regardless of gender, social background, or prior experiences (Xenofontos, 2019). The National Council of Teachers of Mathematics (NCTM) (2008) emphasises that equity does not imply that every student receives equal education; rather, fair and adequate adaptations should be implemented to encourage equal access and accomplishment for all learners. Equity and access are no longer on pages or in the minds of individuals; rather, they are an action-based approach in Mathematics classrooms (NCTM, 2018; Staley, 2020).
In teaching problem-solving and integrating an equitable learning environment, the teacher should put into practice the following: the use of a variety of teaching methods and materials that will meet the diverse needs of every learner in the classroom; the development and design of an engaging assessment for all learners by using different assessment tools and methods; the creation of awareness of cultural diversity in the classroom; the development and incorporation of strong collaborative learning so that each learner will feel a part of the class; and the activation of equal participation in class discussion without any form of discrimination (Gretter et al., 2019; Fuller et al., 2021). McCray (2022) stressed the importance that a Mathematics teacher in an equitable learning environment should demonstrate empathy for all learners, support their learning process, and the development of creativity and critical thinking skills.
The term equity, in the context of Mathematics education, is a fundamental principle that seeks to ensure that all learners have access to quality Mathematics education and can achieve full potential in problem-solving, regardless of background, race, ethnicity, and socio-economic status (Morris & Jacobi, 2022). It implies, for instance, providing resources for needy students so that they can attain the same levels as others. The National Council of Teachers of Mathematics (NCTM, 2008; NCTM, 2018) and (Berry & Larson, 2019) emphasise that equity in Mathematics means having strong and high expectations and wide opportunities for every learner in the classroom setting. Learners with diverse needs have access to learning resources, learning opportunities, and support to enable each learner reach full potential.
Strategies for integrating gender equity in teaching problem-solving
Research evidence shows that female learners’ self-efficacy and attitudes towards Mathematics are strongly influenced by parents, teachers, and peers (UNESCO, 2017; Accenture, 2017; Breda et al., 2020). The findings of these studies made it very important that gender equality be included in problem-solving instruction to guarantee that learners have an equal and independent opportunity to learn and achieve. Radd (2021) presents five (5) practices for equity-focused leadership in the classroom: i) promote a mindset in all members of the class so that each learner will learn at an exceedingly high level; ii) create an inclusive environment that allows learners to feel safe, valued, and cared for; iii) provide all learners access to effective, high-quality curriculum that is both academically rigorous and culturally responsive or relevant; iv) create systems and structures that equitably distribute learning resources;
and v) consider the impact of decision-making and policy on historically marginalised or stereotyped groups.
In line with Radd’s five (5) practices of equity-focused leadership in the teaching and learning of problem-solving, this study identifies key strategies for integrating gender equity in teaching and learning Mathematics, especially problem-solving: i) avoiding gender stereotypes in teaching problem-solving; ii) encouraging all learners’ involvement and collaboration in the teaching and learning process of problem-solving; iii) offering a role model in teaching and learning problem-solving; and iv) allowing self-reflection in Mathematics classrooms. Gender stereotypes are views that Mathematics is a course of study for males, wherein teachers and parents often expect less from female learners in terms of their ability to problem-solving (Acharya et al., 2021). According to researchers, the stereotype is mostly culturally motivated (Fan et al.,2019). Teachers must avoid using gender-biased terminology or instances that perpetuate gender stereotypes in Mathematics classrooms.
In order for teachers to impact the learning experiences of learners, regardless of their gender differences, it is important to use problem-solving situations that include both male and female learners in a range of positions. Ginevra & Nota (2015) emphasised that gender stereotypes should be avoided in the Mathematics classroom since they affect learning and achievement. They added that these characteristics in the classroom can have a negative impact on learners’ self-esteem, school engagement, and competence in Mathematics. It is important to encourage participation in problem-solving activities for all learners. In 2007, the National Centre for Education Research, US Department of Education, highlighted five (5) effective evidence-based practical teaching guides: i) teach girls that academic abilities are expectable and improvable to enhance confidence in their abilities; ii) provide with prescriptive feedback about their performance, focussing on the process of learning, the strategies used during learning and the effort made; iii) expose girls to female role models to challenge negative stereotypes and promote positive beliefs about their abilities; iv) create a classroom environment that sparks curiosity and fosters long-term interest through project-based learning, innovative tasks, and technology; and v) provide opportunities for girls to engage in the learning process. It is important that teachers encourage female learners to pursue Mathematics careers (Halpern et al., 2007).
According to Fuller et al. (2021), teachers who are well-grounded in effective teaching strategies can help promote high levels of motivation and increase self-efficacy and critical thinking abilities in female learners in Mathematics classrooms. Continuous motivation, a strong sense of belonging, and inclusion in all tasks without discrimination, will support gender equity in the classroom, which in turn will promote learners’ self-efficacy, critical thinking skills, and problem-solving skills. Creating an environment that encourages participation in teaching problem-solving is crucial, for it guarantees that all learners are engaged and motivated to learn (Rudhumbu, 2014; UNESCO, 2019). Teachers may be able to encourage classroom participation by employing tactics such as relevant issues, cooperative learning, differentiated teaching, technology, feedback, success celebration, and risk-taking during the teaching and learning process.
Classroom participation strategies encourage learners to solve problems in small groups and promote collaboration, communication, and teamwork. They also promote differentiated instruction, provide different levels of problems, give learners choices, and provide various ways for learners to demonstrate understanding of the concepts. The use of technology further enhances learners’ experiences and makes it easier for teachers to provide feedback; celebrate successes; encourage risk-taking; and provide a safe and supportive environment. Researchers believe that the surest way to create a supportive classroom climate in which all learners feel comfortable sharing their thoughts and opinions, is by promoting productive dialogue among learners in collaborative learning (Gillies, 2019). Teachers are encouraged to avoid ignoring or disparaging learners’ ideas and instead, give positive comments that assist learners in increasing their abilities in solving problems.
METHODOLOGY
This paper is based on a review of journal articles, books, book chapters, and published research using inclusion and exclusion criteria as the method. According to Patino and Ferreira (2018), inclusion and exclusion criteria are method by which research participants are grouped and selected based on certain characteristics that will support the study. Hornberger and Rangu (2020) described this criterion as important to all kinds of research because, according to them, it helps direct the researcher in the selection of respondents that are significant to the study. The researchers used the inclusion and exclusion criteria in selecting journal articles, books, book chapters, and published research based on gender equity in teaching and learning Mathematics, particularly problem-solving. The year of publication of an article was also one of the considerations.
Creswell (2014) outlines a five-step process for analysing data from reviewed articles: i) becoming familiar with the data; ii) coding; iii) developing themes; iv) evaluating; and v) naming these themes. The five (5) steps in the process were summarised into three (3) by Popenoe et al. (2021): i) identifying data that answer the research questions; ii) thematically organising the data; and iii) synthesising, analysing, and presenting the data. The results of the data analysis were compiled and synthesised, in line with the objective of the study.
RESULTS AND DISCUSSION
In this section, the results obtained from the literature review are discussed and presented as strategies identified for integrating gender equity in teaching and learning Mathematics. These strategies are: a) avoid gender stereotypes in the classroom; b) encourage learners’ involvement and collaboration; and c) invite role models to the classroom.
Avoid gender stereotypes in the classroom
Gender stereotypes can limit female learners’ interest in, and ability to solve problems. Studies have shown that gender stereotypes do not only limit female learners in achievement, but also deter female learners from enrolling in courses and choosing careers in Science, Technology, Engineering, and Mathematics (STEM). This has led to under-representation of female learners in STEM careers (Leslie et al., 2015; Meyer et al., 2015). According to Bian et al. (2017), gender stereotypes start as early as six (6) years old and influence female learners’ decisions and abilities in pursuing STEM careers. Law et al. (2021) presented one of the surest ways of avoiding gender stereotypes in the classroom, encouraging an equitable belief system in the classroom. The researchers sampled 143 participants and found that there was gender bias among the male learners. The growth mindset intervention was found to be important for teachers to protect learners from gender stereotypes in the classroom.
Researchers have ascribed the under-representation of female learners in STEM education and careers to high-level gender stereotypes and biases (Meyer et al., 2015; Mitchell & Martin, 2018). Studies have shown how gender stereotypes have affected the learning outcomes of learners, especially female learners, and how they have influenced their choices in STEM careers (Tsouroufli & Redai, 2021). Teachers need to avoid such situations in Mathematics classrooms from an early age of five (5) years (Bian et al., 2017).
Encourage learners’ involvement and collaboration
Mulvey et al. (2020), using the concept of research collaboration, stressed the importance of collaboration in research. Applying this concept to teaching and learning, teachers need to encourage all learners, regardless of upbringing, to get involved and work together. According to McNamee and Moscheta (2015), education is a social construct in which every learner must interact. In a collaborative learning environment, learners learn with fewer gender biases, which in turn promotes equity (Venet, 2021). Therefore, teachers must encourage learners to get involved in the teaching and learning process as well as work in groups as a way of integrating gender equity in Mathematics classrooms.
Invite role models to the classroom
Through invitation of role models to the classroom, teachers help to motivate female learners as well as eliminate or reduce low self-esteem among female learners (Zachmann, 2019). In the process of integrating gender equity in the teaching of problem-solving, teachers should use role-modelling strategies for female learners to show that any learner can pursue any STEM career. According to Burgess et al. (2015), the presence of strong female role models in the classroom has a beneficial effect on the aspirations and academic accomplishments of young women. The researchers use the case of medical education, wherein they emphasise teaching by example, which they attribute to factors that develop learners’ attitudes, values, and professional competencies. The study concluded with the findings that 88% of the respondents perceived teaching by role modelling as an important factor for improved learning. Teaching problem-solving through role modelling is one of the ways to promote gender equity in Mathematics classrooms. Research findings have shown that this is one of the most effective ways of breaking the gender gap in STEM careers as well as the under-representation of female learners in STEM careers (Verdugo-Castro et al., 2021).
It is concluded from this review that gender equity is a key construct to support learning process in Mathematics classrooms, especially problem-solving. The findings are in line with those of Andreasson et al. (2015), in which they emphasized on the importance of pedagogical equity in Mathematics education. According to the definition of gender equity, the underlying truth is that equity in Mathematics education is an integral part of closing the gender gap in STEM education and career paths. Bianchini et al. (2015) consider gender equity as an important discourse that will improve Science, Technology, Engineering, and Mathematics (STEM) education. They recommended the integration of equity in STEM education so that learners can find a safe and conducive environment for making scientific choices and developing critical thinking skills. The findings of Ghosh et al. (2020) support this paper’s conclusion because they concluded that female learners had better attitudes towards gender equity than male learners. The fascinating thing is that they recommended a growing interest in creating continuous and consistent awareness of the importance of gender equity in Mathematics classrooms.
It is imperative that teachers work towards promoting gender equity in Mathematics classrooms by adopting teaching strategies that enhance representation, inclusiveness, empowerment, and equity. The strategies can be implemented by avoiding gender stereotypes, encouraging learners’ involvement and collaboration in the teaching and learning process, and offering role-modelling teaching. According to Moè et al. (2020), gender stereotypes influence learners’ performance on cognitive tasks. Solving word-problems is one of the cognitive tasks, so teachers must avoid gender stereotypes in all forms of teaching and learning Mathematics. This study concludes that the integration of gender equity in the teaching and learning of Mathematics is very important and helps to improve learners’ ability in problem-solving and ultimately better achievement in Mathematics. The researchers recommend further study on strategies for integrating gender equity in Mathematics classrooms.
- Accenture. (2017). Accenture finds girls’ take-up of STEM subjects is held back by stereotypes, negative perceptions and poor understanding of career options. Newsroom.accenture.com. https://newsroom.accenture.com/news/accenture-finds-girls-take-up-of-stem-subjects-is-held-back-by-stereotypes-negative-perceptions-and-poor
- Acharya, B. R., Kshetree, M. P., Khanal, B., Panthi, R. K., & Belbase, S. (2021). Mathematics educators’ perspectives on the cultural relevance of basic level Mathematics in Nepal. Journal on Mathematics Education, 12(1), 17–48. https://doi.org/10.22342/jme.12.1.12955.17-48
- Akala, B. M. (2019). Intersecting human development, social justice and gender equity: A capability option. Education as Change, 23. https://doi.org/10.25159/1947-9417/4080
- Akala, B., & Divala, J. (2016). Gender equity tensions in South Africas post-apartheid higher education: In defence of differentiation. South African Journal of Higher Education, 30(1). https://doi.org/10.20853/30-1-557
- Andreasson, I., Ohlsson, L., & Assarson, I. (2015). Operationalizing Equity: The complexities of equity in practice. Education, Citizenship and Social Justice, 10(3), 266–277. https://doi.org/10.1177/1746197915607280
- Berry, R. Q., & Larson, M. R. (2019). The need to catalyze change in high school Mathematics. Phi Delta Kappan, 100(6), 39–44. https://doi.org/10.1177/0031721719834027
- Bian, L., Leslie, S.-J., & Cimpian, A. (2017). Gender stereotypes about intellectual ability emerge early and influence children’s interests. Science, 355(6323), 389–391. https://doi.org/10.1126/science.aah6524
- Bianchini, J. A., Dwyer, H. A., Brenner, M. E., & Wearly, A. J. (2015). Facilitating Science and Mathematics Teachers’ Talk About Equity: What Are the Strengths and Limitations of Four Strategies for Professional Learning? Science Education, 99(3), 577–610. https://doi.org/10.1002/sce.21160
- Breda, T., Jouini, E., Napp, C., & Thebault, G. (2020). Gender stereotypes can explain the gender equality paradox. Proceedings of the National Academy of Sciences, 117(49), 202008704. https://doi.org/10.1073/pnas.2008704117
- Burgess, A., Oates, K., & Goulston, K. (2015). Role modelling in medical education: The importance of teaching skills. The Clinical Teacher, 13(2), 134–137. https://doi.org/10.1111/tct.12397
- Ergasheva, O. M. Q. (2021). The importance of gender equality in maintaining social justice and women’s contribution to the development of societies. Current Research Journal of History, 02(06), 49–52. https://doi.org/10.37547/history-crjh-02-06-11
- Fan, Y., Shepherd, L. J., Slavich, E., Waters, D., Stone, M., Abel, R., & Johnston, E. L. (2019). Gender and cultural bias in student evaluations: Why representation matters. PLOS ONE, 14(2), e0209749. https://doi.org/10.1371/journal.pone.0209749
- For, O. (2021). Adapting curriculum to bridge equity gaps: Towards an inclusive curriculum. Oecd Publishing.
- Fuller, J. A., Luckey, S., Odean, R., & Lang, S. N. (2021). Creating a diverse, inclusive, and equitable learning environment to support children of colour’s early introductions to STEM. Translational Issues in Psychological Science, 7(4), 473–486. https://doi.org/10.1037/tps0000313
- Ghosh, P., Jha, S., Dasgupta, A., Paul, B., & Biswas, A. (2020). Attitude and perception of gender equity among learners and teachers of a rural school in west Bengal: A mixed-method approach. Journal of Education and Health Promotion, 9(1), 330. https://doi.org/10.4103/jehp.jehp_597_20
- Gillies, R. M. (2019). Promoting academically productive student dialogue during collaborative learning. International Journal of Educational Research, 97, 200–209. https://doi.org/10.1016/j.ijer.2017.07.014
- Ginevra, M. C., & Nota, L. (2015). Occupational gender stereotypes and problem-solving in Italian adolescents. British Journal of Guidance & Counselling, 45(3), 312–327. https://doi.org/10.1080/03069885.2015.1063584
- Gretter, S., Yadav, A., Sands, P., & Hambrusch, S. (2019). Equitable learning environments in K-12 computing. ACM Transactions on Computing Education, 19(3), 1–16. https://doi.org/10.1145/3282939
- Halpern, D., Aronso, J., Reimer, N., Simpkins, S., Star, J., & Wentzel, K. (2007). Encouraging girls in Mathematics and science (NCER 2007 – 2003). Washington DC, National Center for Education Research, Institute of Education Science, US Department of Education.
- Hornberger, B., & Rangu, S. (2020). Designing inclusion and exclusion criteria. https://repository.upenn.edu/crp/1
- Idin, S., & Donmez, I. (2017). The views of Turkish science teachers about gender equity within science education. Science Education International, 28(2), 119–127. https://doi.org/10.33828/sei.v28.i2.4
- Law, F., McGuire, L., Winterbottom, M., & Rutland, A. (2021). Children’s gender stereotypes in STEM following a one-shot growth mindset intervention in a science museum. Frontiers in Psychology, 12. https://doi.org/10.3389/fpsyg.2021.641695
- Leslie, S.-J. ., Cimpian, A., Meyer, M., & Freeland, E. (2015). Expectations of brilliance underlie gender distributions across academic disciplines. Science, 347(6219), 262–265. https://doi.org/10.1126/science.1261375
- Mawarti, S., & Nurlaelah, E. (2020). Social justice in Mathematics education for sustainable development. Journal of Physics: Conference Series, 1521(3), 032094. https://doi.org/10.1088/1742-6596/1521/3/032094
- McNamee, S., & Moscheta, M. (2015). Relational intelligence and collaborative learning. New Directions for Teaching and Learning, 2015(143), 25–40. https://doi.org/10.1002/tl.20134
- Meyer, M., Cimpian, A., & Leslie, S.-J. (2015). Women are underrepresented in fields where success is believed to require brilliance. Frontiers in Psychology, 6. https://doi.org/10.3389/fpsyg.2015.00235
- Mitchell, K. M. W., & Martin, J. (2018). Gender bias in student evaluations. PS: Political Science & Politics, 51(03), 648–652. https://doi.org/10.1017/s104909651800001x
- Moè, A., Hausmann, M., & Hirnstein, M. (2020). Gender stereotypes and incremental beliefs in STEM and non-STEM learners in three countries: Relationships with performance in cognitive tasks. Psychological Research, 85(2). https://doi.org/10.1007/s00426-019-01285-0
- Morris, H., & Jacobi, L. (2022). It takes the university to close the equity gap. The International Journal of Equity and Social Justice in Higher Education, 1(1). https://doi.org/10.56816/2771-1803.1006
- Mulvey, K. L., McGuire, L., Hoffman, A. J., Hartstone‐Rose, A., Winterbottom, M., Balkwill, F., Fields, G. E., Burns, K., Drews, M., Chatton, M., Eaves, N., Law, F., Joy, A., & Rutland, A. (2020). Learning hand in hand: Engaging in research–practice partnerships to advance developmental science. New Directions for Child and Adolescent Development, 2020(172), 125–134. https://doi.org/10.1002/cad.20364
- National Council of Teachers of Mathematics (NCTM). (2008). Principles and standards for school Mathematics. National Council of Teachers of Mathematics; Portland, Oregon.
- National Council of Teachers of Mathematics (NCTM). (2018). Catalysing change in high school Mathematics: Initiating critical conversations.
- Patino, C. M., & Ferreira, J. C. (2018). Inclusion and exclusion criteria in research studies: Definitions and why they matter. Journal Brasileiro de Pneumological, 44(2), 84. NCBI.
- Popenoe, R., Langius-Eklöf, A., Stenwall, E., & Jervaeus, A. (2021). A practical guide to data analysis in general literature reviews. Nordic Journal of Nursing Research, 41(4), 175–186. Sagepub. https://doi.org/10.1177/2057158521991949
- Radd, S. I. (2021). Five practices for equity-focused school leadership. ASCD.
- Rodríguez A. J., & Suriel, R. L. (2022). Equity in STEM education research: Advocating for equitable attention. Springer.
- Rodríguez Martínez, S., Regueiro Fernández, B., Piñeiro Aguín, I., Estévez Blanco, I., & Valle Arias, A. (2020). Gender differences in Mathematics motivation: Differential effects on performance in primary education. Minerva.usc.es, 10. https://doi.org/10.3389/fpsyg.2019.03050
- Rudhumbu, N. (2014). Motivational strategies in the teaching of primary school Mathematics in Zimbabwe. International Journal of Education Learning and Development UK, 2(2), 76–103.
- Spielman, L. J. (2008). Equity in Mathematics education: Unions and intersections of feminist and social justice literature. ZDM, 40(4), 647–657. https://doi.org/10.1007/s11858-008-0113-0
- Staley, J. W. (2020). Then: Equity and access. Mathematics Teacher: Learning and Teaching PK-12, 113(9), 759–761. https://doi.org/10.5951/mtlt.2020.0080
- Tsouroufli, M., & Redai, D. (Eds.). (2021). Gender equality and stereotyping in secondary schools. https://doi.org/10.1007/978-3-030-64126-9
- Ty, R. (2011). Social injustice, human rights-based education and citizens’ direct action to promote social transformation in the Philippines. Education, Citizenship and Social Justice, 6(3), 205–221. https://doi.org/10.1177/1746197911417413
- UNESCO. (2015). Education for all 2000-2015: Achievements and challenges. United Nations Educational, Scientific And Cultural Organization.
- UNESCO. (2017). Cracking the code: Girls’ and women’s education in science, technology, engineering and Mathematics (STEM). https://doi.org/10.54675/qyhk2407
- UNESCO. (2019). Building bridges for gender equality. Unesco.
- UNESCO. (2022). Global education monitoring report: Gender report, deepening the debate on those still left behind. UNESCO; Sustainable Development Goals; Global Education Monitoring Report.
- Vale, C., Atweh, B., Averill, R., & Skourdoumbis, A. (2016). Equity, social justice and ethics in Mathematics education. Research in Mathematics Education in Australasia 2012-2015, 97–118. https://doi.org/10.1007/978-981-10-1419-2_6
- Vale, C., Averill, R., Hall, J., Forgasz, H., & Leder, G. (2020). Equity, social justice, and ethics. Research in Mathematics Education in Australasia 2016–2019, 177–208. https://doi.org/10.1007/978-981-15-4269-5_8
- Venet, A. S. (2021). Equity-centred trauma-informed education. W.W. Norton And Company.
- Verdugo-Castro, S., García-Holgado, A., Sánchez-Gómez, M. C., & García-Peñalvo, F. J. (2021). Multimedia analysis of Spanish female role models in science, technology, engineering and Mathematics. Sustainability, 13(22), 12612. https://doi.org/10.3390/su132212612
- Wang, M.-T., & Degol, J. L. (2016). The gender gap in Science, Technology, Engineering, and Mathematics (STEM): Current knowledge, implications for practice, policy, and future directions. Educational Psychology Review, 29(1), 119–140. https://doi.org/10.1007/s10648-015-9355-x
- Zachmann, K. (2019). Women in STEM: Female role models and gender-equitable teaching strategies. Stkate.edu. https://sophia.stkate.edu/maed/
Article Statistics
Track views and downloads to measure the impact and reach of your article.
PDF Downloads
Subscribe to Our Newsletter
Sign up for our newsletter, to get updates regarding the Call for Paper, Papers & Research.
Email Address * Subscribe
Track Your Paper
Enter the following details to get the information about your paper
A RESOURCE CREATED BY:
How Partner Gender Influences Female Students’ Problem Solving in Physics Education
During peer learning activities in physics class, female students paired with other females learn better than those paired with males..
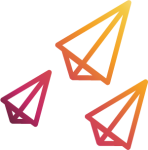
Cite this Article
Ding, Ning, and Egbert Harskamp. "How partner gender influences female students’ problem solving in physics education." Journal of Science Education and Technology 15.5-6 (2006): 331-343.
Ding, N., & Harskamp, E. (2006). How partner gender influences female students’ problem solving in physics education. Journal of Science Education and Technology , 15 (5-6), 331-343.
Ding, Ning, and Egbert Harskamp. "How partner gender influences female students’ problem solving in physics education." Journal of Science Education and Technology 15, no. 5-6 (2006): 331-343.
Egbert Harskamp
- Introduction
- Methodology
Grouping students into pairs to solve problems can be a powerful learning tool. Peer learning can motivate students, enhance critical thinking skills, and enable students to transform classroom information into working knowledge. It promotes students to order their thoughts coherently, to understand the ideas and questions of others, and to solve problems through interpersonal interactions. The gender of students’ partners during these activities, however, can sometimes negatively affect their learning outcomes given that females and males oftentimes have different communication styles. Prior research has shown that females are more likely to initiate conversation by asking questions while males begin discussions by ‘‘presenting explanations.’’ In the field of physics, such differences in communication styles may be one of the reasons that female students are often unable to benefit as much as male students from cooperative learning exercises. While there is no clear empirical evidence as to whether female students’ interaction style and problem-solving processes are influenced by their partner gender, it is assumed that the females’ lack of self-confidence in physics will exacerbate underlying communication difficulties in mixed-gender partnerships. This study explores how pairing students based on gender can influence students’ test scores and problem solving skills in physics.
In Shanghai, female high school students paired with male students performed worse when solving physics problems than those paired with other females.
- Female students who were paired with male students during a cooperative learning exercise were at a significant disadvantage when comparing their performance on a physics exam before and after participating in the group work. Females who were paired with males did significantly worse on the post-test examination than did females who were paired with other females, and on average, scored 2 points worse.
- Communication differences emerged between men and women during cooperative learning exercises. Females paired with males were significantly less likely than females paired with females to offer problem-related information (67 times versus 150 times) or give suggestions on how to solve the problem (22 times versus 54 times), which amounted to a difference of 22 times versus 54 times during group exercises. Additionally females showed a significantly higher degree of uncertainty and generated more agreements than did their male partners. Compared with their male partners, female
- All female pairs performed just as well as their male classmates when solving problems and taking tests. Additionally, they demonstrated confidence, put forward their ideas freely, actively participated in problem solving, and cooperated with their partners. Specifically, in the mixed-gender groups, 18% of messages from females concerned strategizing a solution, whereas 34% did from the all-female group; and for the all-female groups, 8% of messages centered on executing on the chosen plan, while only 3% of messages focused on this in the mixed-gender group.
- While females are more affected by their partner’s gender when working in pairs, male test scores and problem-solving skills are not influenced by their partner’s gender. In the male-male pairings, the only statistical difference appeared in messages around executing a plan. In mixed groups, 11% of males’ messages were about executing a plan, compared to only 1% in the same-gender group.
In short, gender plays an important role in girls’ learning outcomes when they participate in peer activities. Female students are more likely to be active participants in problem solving exercises and excel in test taking when paired with other females.
This study randomly paired high school students to complete a physics assignment in all-male, all-female, or mixed-gender pairs as part of a cooperative learning exercise. While solving the problem, a student could only communicate with his or her partner by writing on a blank piece of paper, which recorded their dialogue. Students took tests on the subject matter before and after the exercise to determine their problem-solving capabilities. Additionally, students’ communication-log sheets were used for analysis of communication style and of the content of the written messages. This study included 50 high school students (26 females and 24 males) from two 11 th grade physics classes in Shanghai. The average student age was 16.
- Top of Page
- Authors & Citations
- Related Studies
Related GAP Studies
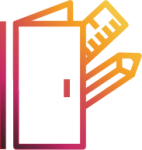
A cash transfer program in Kenya significantly boosted school enrollment among adolescent girls and elevated expectations for completing secondary education.
Effects of a single cash transfer on school re-enrollment during covid-19 among vulnerable adolescent girls in kenya: randomized controlled trial.
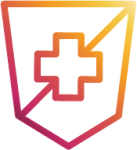
Peer-led sex education significantly boosts contraceptive use and demand while reducing unmet contraceptive needs among Ethiopian adolescent girls.
Effectiveness of peer-led education interventions on contraceptive use, unmet need, and demand among adolescent girls in gedeo zone, south ethiopia. a cluster randomized controlled trial.
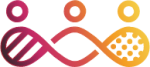

Seven exercises to incorporate into your gender studies classes
It can be challenging to come up with new and interesting ways to educate your students on gender issues. Eunice Costilla Cruz outlines seven ways

Eunice Costilla Cruz
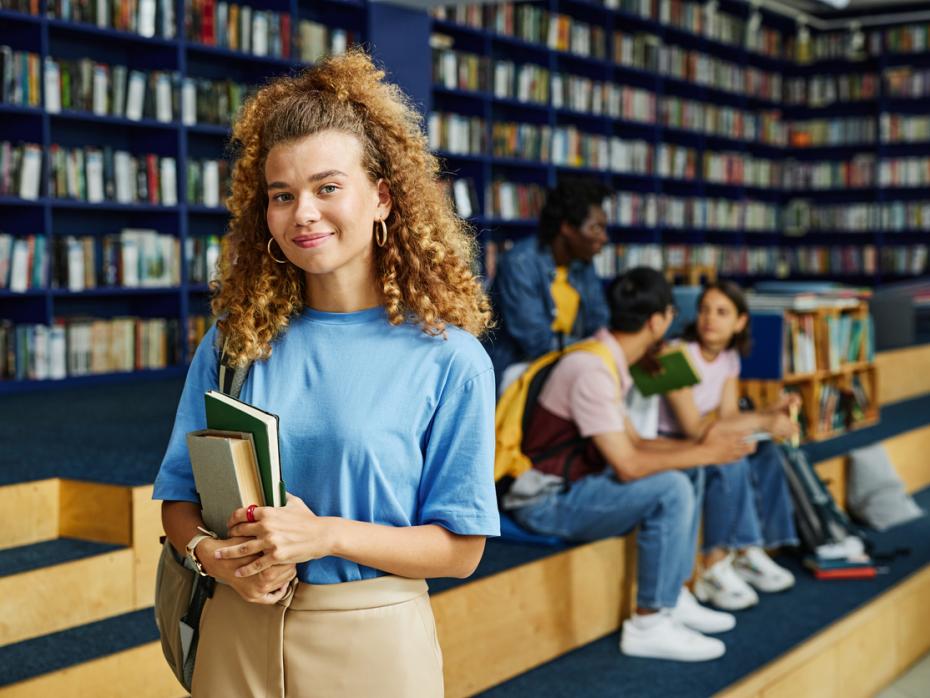
Created in partnership with
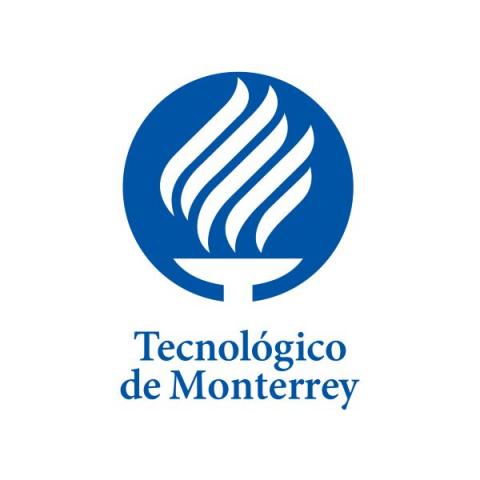
You may also like
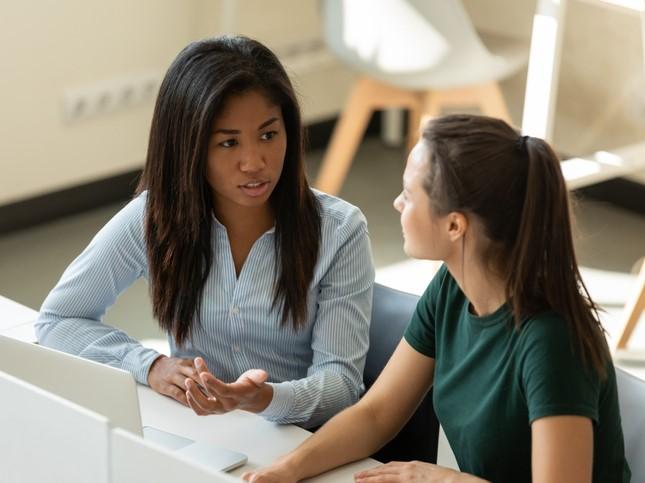
Popular resources
.css-1txxx8u{overflow:hidden;max-height:81px;text-indent:0px;} Students using generative AI to write essays isn't a crisis
How students’ genai skills affect assignment instructions, turn individual wins into team achievements in group work, access and equity: two crucial aspects of applied learning, emotions and learning: what role do emotions play in how and why students learn.
As well as being spaces for learning and generating knowledge, universities are also sites for reflecting on and generating awareness about contemporary social issues, such as gender inequality. We must take our duty to encourage dialogue seriously to nurture responsibility and accountability in our students. Here are six strategies.
Create online learning communities
Using Flip , a video-discussion platform is a great way to promote dialogue between students on important topics. Students can communicate by creating short videos on a concept or case study related to gender inequality that they have encountered in class. I suggest starting this activity with a trigger question, including instructions, responding to questions and later asking students to give feedback on the exercise. This practice will build a collaborative learning environment that helps students develop an understanding of important issues.
Bring learning to life with immersive 360° videos
360° videos, in which students can view and virtually explore content generated from real situations, offer experiential learning opportunities. Not all institutions have the resources to create their own virtual tours, so if you can’t, you can search for free videos online and plan activities that focus on a specific gender issue, such as stereotypes, discrimination or harassment.
Encourage students to interview affected individuals
Encourage your students to conduct interviews with family members from a different generation to identify changes in attitudes to gender roles and experiences of discrimination and find out how they affected their lives. After their interviews, students can outline challenges still relevant today and their social implications. For this activity, I recommend providing the students with resources on the topic and on how to design effective questions.
- Resource collection: Gender equality in higher education: how to overcome key challenges
- Mentoring and teaching women with disabilities in universities
- Are STEM admissions processes hindering our diversity efforts?
Use cinema as a didactic resource
Integrating films, documentaries, short films and other audiovisual resources into course material can help students identify stereotypes, generate historical awareness, strengthen empathy, understand social issues and develop critical thinking. Examples of activities to try with your students include film analysis, comparative work and panel discussions. You can also organise film debate sessions involving the entire course community. I recommend that you anticipate possible technical problems such as access restrictions or issues with the streaming platform before holding these sessions.
Bring out your students’ creativity
Prompting your students to develop creative assets such as infographics, posters, comics and brochures responding to a relevant topic can motivate them to become engaged in solving gender-related issues (such as violence or exclusionary language). Digital tools can be your allies, here, as several applications and websites make it easier for your students to design these resources. Examples include Canva, Piktochart , Adobe Spark and Easel.ly . Guide your students throughout this exercise, pointing out the goal (for example, to work towards the elimination of gender violence, discrimination or inequalities) and make the importance of generating creative solutions clear.

Organise sessions with experts in the field
Schedule virtual or face-to-face sessions in which experts in gender studies and human rights can present their experiences and achievements in advancing gender equality. You can reach out to activists, academics and members of marginalised communities to ask them to share their stories, their influences and their upcoming projects. Find them at your university, non-profit organisations, academic events, professional networking sites and elsewhere. The sessions can take the form of conferences, talks, interviews, panel discussions, debates or workshops/seminars. Regardless of the format, aim to facilitate ideas exchange between the students and the speaker/s.
Set a ‘women in history’ research project
Exploring the lives and experiences of women throughout history can promote historical awareness, empathy and understanding of gender disparity. Advise your group on conducting research using reliable sources and ask them to submit their work in the form of a presentation, video, virtual gallery, posters, podcasts or even digital magazine.
I hope that these exercises will raise your students’ awareness of gender issues and enhance engagement. Remember that, before implementing any of these activities, you must establish your objectives, gain an understanding of your student demographics and decide on the format. I also recommend that, once the activity is over, you use polls and surveys to gauge the efficacy of your efforts and make adjustments as necessary.
Eunice Costilla Cruz is tutor professor of humanities and social sciences at Monterrey Institute of Technology, Mexico.
If you would like advice and insight from academics and university staff delivered direct to your inbox each week, sign up for the Campus newsletter .
Students using generative AI to write essays isn't a crisis
Eleven ways to support international students, indigenising teaching through traditional knowledge, seven exercises to use in your gender studies classes, rather than restrict the use of ai, embrace the challenge, how hard can it be testing ai detection tools.
Register for free
and unlock a host of features on the THE site

An official website of the United States government
The .gov means it’s official. Federal government websites often end in .gov or .mil. Before sharing sensitive information, make sure you’re on a federal government site.
The site is secure. The https:// ensures that you are connecting to the official website and that any information you provide is encrypted and transmitted securely.
- Publications
- Account settings
Preview improvements coming to the PMC website in October 2024. Learn More or Try it out now .
- Advanced Search
- Journal List
- Front Psychol
Gender Differences in Visuospatial Abilities and Complex Mathematical Problem Solving
Isabel m. ramírez-uclés.
1 Department of Psychology of Personality, Evaluation and Psychological Treatments of the National University of Distance Education, Madrid, Spain
Rafael Ramírez-Uclés
2 Department of Mathematics Education at the University of Granada, Granada, Spain
Associated Data
The datasets generated for this study are available on request to the corresponding author.
Mathematical problem-solving and spatial visualization are areas in which performance has been shown to vary with sex. This article describes the impact of gender on spatial relations measured in 331 secondary school students (202 males, 129 females), 145 (105 males, 40 females) of whom had been selected to participate in a mathematical talent stimulation project after passing a complex problem-solving test. In the two tests administered, the Differential Aptitude Tests-Space Relations ( DAT-SR ) and the Primary Mental Abilities-Space Relations ( PMA-SR ), performance was assessed on the grounds of both absolute scores and the ratio to the number of items answered. The students participating in the talent program earned higher scores on both tests, although no interaction was identified between mathematical abilities and gender in connection with the differences in spatial habilities observed. In PMA-SR, boys answered more items and scored higher, whereas in DAT-SR girls tended to omit more items. None of the indicators studied exhibited differences between the sexes in both tests and in some cases the differences in the absolute values of the indicators were absent when expressed as ratios.
Introduction
Although the importance of visualization in mathematical problem solving has been highlighted in mathematics education ( Clements and Battista, 1992 ; Arcavi, 2003 ), no consensus has yet been reached on its role in improving performance ( Bishop, 1980 ; Lean and Clements, 1981 ). Traditional studies concluded that spatial awareness and the capacity to visualize abstract mathematical relationships were not necessarily components of mathematical talent ( Krutetskii, 1976 ), whilst later studies revealed that talented students preferred non-visual methods ( Presmeg, 1986 ). More recent research has found significant evidence of a relationship between visualization and mathematical ability, however ( Rivera, 2011 ; Rabab’h and Veloo, 2015 ; Ramírez and Flores, 2017 ). The controversial findings are explained by the existence of different conceptions of mathematical talent and visualization, thereby requiring a clear view on what factors are used in the research to characterize both mathematical talent and visualization. Although there is consensus in that visualization should be considered an inherent ability needed to accomplish certain mathematical tasks, there is still no consensus on what instruments are most appropriate for identification of mathematical talent ( Pitta-Pantazi and Christou, 2009 ).
A number of studies has focused on gender differences in these two areas, suggesting possible relationships between them ( Ganley and Vasilyeva, 2011 ). Gender differences in spatial skills may serve as cognitive predictors of mathematical performance, particularly as regards geometry. Gender differences in spatial reasoning, together with the partial contribution of visual reasoning to problem solving, may have gender-related implications in mathematical contexts. Whilst males and females differ in spatial visualization and performance in high school geometry, however, their logical reasoning skills and use of geometric problem-solving strategies are indistinguishable ( Battista, 1990 ).
This exploration of the effect of gender and mathematical performance on the differences observed in secondary school students’ visual abilities includes a review of the literature on gender differences in the two types of skills.
Gender Differences in Mathematical Performance
Review papers and meta-analyses have identified greater mathematical problem-solving aptitudes among men ( Maccoby and Jacklin, 1974 ; Hyde et al., 1990 ; Hyde, 2014 ). Hyde et al. (1990) reported wider differences between male and female secondary school students in complex problem solving than in parameters such as computation or understanding mathematical concepts. They observed no gender difference in arithmetic or algebra. Male superiority in geometry was minor, whilst the widest gender gap was recorded for tests with mixed content. The exercises used to assess mathematical performance have also been deemed to affect the results, with men performing better than women in problems involving mathematical reasoning ( Halpern, 2000 ) and word problems which to be solved must be translated into mathematical terminology ( Low and Over, 1993 ).
Other factors to be considered in gender difference studies is the date they are conducted and the group of people participating. A meta-analysis conducted 18 years later by Hyde et al. (2008) with 2nd to 11th year students in the United States revealed no difference between boys’ and girls’ lower level mathematical skills. When items entailing complex problem solving were included, girls in year 12 performed as well as their male classmates. Similarly, in a meta-analysis of studies conducted from 1990 to 2007, Lindberg et al. (2010) found only a minor difference between the sexes in complex problem solving. Else-Quest et al. (2010) conducted a meta-analysis of gender differences in mathematical performance, reporting substantial inter-country variability while also furnishing further evidence that, on average, males and females differ vary little in mathematics achievement, despite more positive attitudes toward mathematics among the former.
Although women continue to be underrepresented in science, technology, engineering and mathematics (STEM) education and careers ( Else-Quest et al., 2013 ), gender differences in mathematical performance have been less consistently found ( Ganley and Vasilyeva, 2011 ). Unlike other meta-analyses of performance in mathematical tests that reported males to perform more highly than females, a study on classroom gender differences authored by Voyer and Voyer (2014) found women to earn higher marks in all areas. That variability can be attributed to the diversity of the instruments used to measure mathematical performance ( Gibbs, 2010 ). Boys have been perceived to be academically stronger in mathematics and science ( Olszewski-Kubilius and Turner, 2002 ), with more male than female high-achievers in those subjects ( Reis and Park, 2001 ). Gender differences have been recorded in tests assessing mathematical talent in students aged 12 to 14 ( Benbow and Stanley, 1996 ). The decline in the male-female ratio among the highest scoring students in recent years calls for further study, however. Some authors have suggested that the male advantage in mathematical skills may be limited to the upper end of the talent distribution ( Halpern et al., 2007 ).
Further to a meta-analysis of differences between the sexes in mathematics covering a number of countries ( Else-Quest et al., 2010 ), the largest mean effect size was recorded in the PISA ( Program for International Student Assessment ) space/shape domain, which assesses the understanding of spatial relationships. The population studied, students aged 14 to 16, was deemed old enough to be able to solve complex mathematical problems. The data for that meta-analysis were drawn from the 2003 TIMSS ( Trends in International Mathematics and Science Study and the Program for International Student Assessment ), however, in which Spanish students did not participate. This study aims to further investigate on this particular group of students.
Gender Differences in Visuospatial Ability: Performance Factors
Meta-analyses have consistently reported males to be more spatially skilled than females ( Linn and Petersen, 1985 ; Hedges and Nowell, 1995 ; Voyer and Saunders, 2004 ; Halpern et al., 2007 ). A host of authors ( Strand et al., 2006 ; Steinmayr and Spinath, 2008 ; Voyer and Voyer, 2014 ; Wach et al., 2015 ) has observed men to score higher than women on visuospatial tests, in particular in connection with mental rotation, where several authors observed a wider gap between men’s and women’s scores than in skills such as spatial perception or visualization ( Voyer et al., 1995 ; Alansari et al., 2008 ; Geiser et al., 2008 ; Moè, 2009 ; Hyde, 2014 ; Xu et al., 2016 ). This study aims to look deeper into the prevalent role of mental rotation in gender differences, hence we will compare the results of a mental rotation test with those of another test related to the spatial ability of visualizing an object in three dimensions from a two-dimensional model.
Different performance factors have been identified in the effect of gender on mental rotation results, depending on the measuring instrument used and the conditions in which the tests were administered and scored. In a 3D mental rotation test measuring speed of performance as one such factor, time limits and the use of raw scores were found to benefit males ( Goldstein et al., 1990 ). Loring-Meier and Halpern (1999) found males to answer more rapidly than females, whereas no difference was observed between them in the number of correct answers to items unrelated to mental rotation but involving visuospatial working memory. Robert and Chevrier (2003) reported similar numbers of correct answers among men and women when no time limit was established in mental rotation test, although men answered the items more quickly than women. Whilst some studies showed that such gender differences are more pronounced when the time to do the test is limited in mental rotation test ( Voyer and Saunders, 2004 ; Peters, 2005 ; Voyer, 2011 ; Maeda and Yoon, 2016 ), others designed to assess mental rotation aptitudes reported no statistically significant differences between the sexes in completion time ( Yoon and Mann, 2017 ). A third group observed males to score higher on visual tests irrespective of the existence of time limitations in mental rotation test ( Delgado and Prieto, 1996 ; Geiser et al., 2006 ) or other figure analogy test ( Blum et al., 2015 ). The use of ratios to score mental rotation performance significantly narrowed gender-related differences ( Stumpf, 1993 ), whereas that approach reduced the gap between the two sexes’ scores for other aptitudes less meaningfully. Subsequent studies questioned the effect of these factors, confirming that the raw score-measured effect size of gender differences was unaffected when longer test times were allowed and that the reluctance to guess was similar for males and females, while males answered correctly to more exercises irrespective of timing ( Delgado and Prieto, 1996 ). Masters (1998) found no evidence that the gender differences in mental rotation tests were affected by the scoring method or the time limit, with men scoring higher than women regardless of the scoring procedure. Other authors reported that the magnitude of gender differences in mental rotation was similar in distinct timing conditions when a conventional scoring method was used ( Voyer et al., 2004 ).
The effect of time is associated with the strategy used to complete tests, with women being shown to be less self-assured when sitting these tests in mental rotation ( Cooke-Simpson and Voyer, 2007 ) or in questionnaires about attitude and belief ( Parsons et al., 1982 ) and as a result to adopt more conservative strategies in mental rotation test of other test ( Hong and Aqui, 2004 ; Voyer and Saunders, 2004 ; Hirnstein et al., 2009 ). Research in figure analogy test has found women to be slower to answer and more reluctant to guess at answers in items they deem difficult, and hence to leave more items blank than males ( Blum et al., 2015 ). A study of the impact of response latency, response frequency and time invested on a dynamic spatial test revealed that males outperformed females even when the effects of those performance factors were partial ( Contreras et al., 2007 ). Other factors that may attenuate gender differences in mental rotation have also been identified, such as using the ratio of the correct to the attempted items as an alternative scoring criterion ( Goldstein et al., 1990 ).
Purpose of Study
The literature review conducted for this article revealed wider differences between the sexes in mental rotation than other spatial exercises. No consensus was detected, however, on how such differences may be impacted by scoring criteria, i.e., by the use of absolute values or the ratio of each to the number of items answered. The review also identified the early years of secondary school as the time when gender differences appear in complex mathematical problem solving. No conclusive evidence was found of interaction between spatial skills and complex problem-solving abilities in the differences between the sexes observed, particularly among Spanish students.
With a view to contributing to this issue, the research questions posed in this study were: do gender and the ability to solve complex problems affect the differences observed in the participants of the current study’ spatial aptitudes? If so, what performance measurements reflect that effect? To this end, results of 13- to 16-year old Spanish students are compared in two different test assessing the spatial ability (mental rotation and visualization of an object in three dimensions from a two-dimensional model) as well as the factors related to performance, completion time, and strategies used to answer the items.
Materials and Methods
A total of 331 s, 2nd, 3rd and 4th -year secondary education students participated in this study. The mean age of the sample was 15 (±0.97) and the range 13 to 16. Part of the sample, 105 males and 40 females from nine provinces in Spain, were selected to participate in ESTALMAT, a project to encourage mathematically talented students, selected on the grounds of a math test in which the problems were divided into sections by level of difficulty. The participants didn’t receive any incentives. The test assessed students’ aptitude for and attitudes around mathematical knowledge. The differences in the number of boys and girls in this group attested to the differences between the sexes in complex problem-solving reported for youths of those ages, especially where the questionnaires combined areas such as geometry, arithmetic and logical reasoning ( Hyde et al., 1990 ; Hyde, 2014 ). These students (‘’) had proven their ability to solve complex mathematical problems by passing a test with problems such as the following.
‘The vertices of a triangle bear the number 1 or −1 and the product of the three is shown in the middle. If we add the four numbers: (a) What values may the sum take? What combination yields zero? (b) What would the sum be if instead of a triangle we had a square? (c) If we use a polygon with an even number of sides, can the sum be zero? Why? (d) What sort of polygons with an odd number of sides could give us zero? Why?’
The 186 students (97 males and 89 females) in the other group were enrolled in 2nd, 3rd, or 4th-year secondary education in two schools, each in a different Spanish province. According to their teachers, these students (‘NCPs’) had exhibited no complex problem-solving talent.
With a view to exploring the issue in greater depth, this study analyzed the effect of gender and mathematical ability on performance in two spatial tests frequently used to diagnose spatial aptitudes in Spain.
The following instruments were used in this study:
- - The Primary Mental Abilities Test (PMA) – Spatial Relations (SR) ( Thurstone and Thurstone, 1976 ). Thurstone’s initial battery of PMA tests yielded seven ‘primary mental abilities’: verbal comprehension (V), spatial orientation (S), inductive reasoning (I or R), number (N), word fluency (W), associative memory (M), and perceptual speed (P). The Spanish adaptation was created by TEA Ediciones in 1987. This study applied the test for spatial relations, defined in the Spanish edition as ‘the ability to interpret and recognize objects that change their spatial position, while maintaining their internal structure’. Cronbach’s alpha (a measure of reliability or internal consistency) for the SR factor has been shown to be 0.93, whilst the value calculated for the present sample was 0.89.
PMA-SR measures the ability to mentally rotate two-dimensional figures quickly and accurately ( Linn and Petersen, 1985 ; Voyer, 2011 ). One of its features favored by researchers is the correction for guessing, for the final score is the number of correct minus the number of incorrect answers ( Voyer and Saunders, 2004 ). Another prominent characteristic is the short time allowed, just 5 min, to answer 20 multiple-choice items, each with six options. Subjects consequently have an average of 15 s to analyze the six options in each item, without knowing how many are correct. Differences between the sexes have been identified for PMA-SR, with men scoring higher ( Stericker and LeVesconte, 1982 ; Kail et al., 1984 ; Campos, 2014 ).
- - The Differential Aptitude Test (DAT-5) – Space Relations (SR) ( Bennett et al., 2000 ). The tests in the fifth version of the DAT assess eight aptitudes: verbal, numerical and abstract reasoning, perceptual speed and accuracy, mechanical reasoning, space relations and spelling and language usage. The Spanish adaptation of the original version was created in 2000 by TEA Ediciones. Level 1 of the space relations (SR) scale was chosen in this study to measure the ability to visualize an object in three dimensions from a two-dimensional model and mentally rotate the object in space. Cronbach’s alpha for groups participating in SR test Level 1 range from 0.86 to 0.93, whilst the value calculated for the present sample was 0.97.
Each test item consists in a two-dimensional drawing, which subjects must match to only one of four three-dimensional figures. This test is often used to study gender differences ( Hartlage, 1970 ; Feingold, 1988 ; Delgado and Prieto, 1996 ), which have been identified by some authors ( Hall, 1979 ) and reported by others to be minor only and less accentuated than observed with the mental rotation test ( Linn and Petersen, 1985 ; Voyer et al., 1995 ; Kaufman, 2007 ). In this test subjects are given 20 min to choose one of four possible replies to each of 50 items. They must consequently answer each item in an average 24 s, although not all four choices must necessarily be analyzed, for participants know only one is correct.
Hereafter, the two aforementioned tests are referred to as PMA-SR and DAT-SR. The working hypothesis defined to explore the impact of gender differences and mathematical abilities on performance indicators was based on the earlier findings described above. The PMA-SR test was therefore deemed more appropriate to detect gender differences in spatial ability, for it measures mental rotation in a specific plane, whereas the DAT-SR test measures the ability to construct a three-dimensional object from its two-dimensional representation. The PMA-SR test might better identify gender differences in speed-related factors, given the short time afforded subjects to complete the exercise. The DAT-SR test, in turn, might furnish a more reliable measure of strategy-based self-confidence. Since there is only one correct answer to each item in DAT-SR, items left blank are a more sensitive indication of student uncertainty and therefore their level of self-confidence. More self-confident subjects would not need to analyze all the options as intensely and could consequently answer more quickly without leaving items blank.
The tests were administered to the original recommendations on instructions and timing. The talented complex problem-solvers sat the tests during one of their ESTALMAT project sessions, routinely conducted outside class time (on Saturday mornings). The PMA-SR instructions were delivered in 5 min, after which students were allowed 5 min to complete the test. After a 30 min break, the DAT-SR test was administered, again with a 5 min explanation followed in this case by 20 min to do the exercise. The same procedure was deployed with the control group students, who participated during normal classroom time.
As students were given no prior information about the scoring procedure, they did not know that the total score in PMA-SR was found as the difference between the number of correct and incorrect answers and in DAT-SR as the number of correct responses. They were, however, told that the number of correct choices per item in PMA-SR was indeterminate and that there was only one per item in DAT-SR.
All the subjects gave their consent to voluntarily participate in the study, which are compliant with the guidelines given by the Bioethics Committee from both UNED and University of Granada in relation to human subjects.
Design and Variables
A 2 × 2, bi-factorial intergroup design was used, in which Gender (categories: male and female) and Ability (categories: CP, talented complex problem-solvers; and NCP, no complex problem-solving talent) were the independent variables. The dependent variables were performance, speed and confidence, measured in terms of the following indicators.
- • Number of correct items (A1): in PMA-SR an item was deemed correct only if, of the six options given, all the actual rotations and no others were chosen. In DAT-SR an item was deemed correctly answered if the single correct option was chosen.
- • Number of incorrect items (A2): in PMA-SR an item was deemed incorrect if any actual rotation was not chosen, or any non-rotations were. In DAT-SR, items were deemed incorrect when the wrong option was chosen.
- • Number of items attempted (B1): the number of items attempted was the number answered: B1 = A1 + A2.
- • Number of blank items (C1): blank items were all the ones where students chose none of the options. In PMA-SR, B1 + C1 = 20 and in DAT-SR, B1 + C1 = 50.
- • Test score (A3): in PMA-SR the score was found by subtracting the number of incorrect from the number of correct items. In DAT-SR the score was the number of correctly answered items.
- • Last item answered (B2): as the items were sorted correlatively, the value was the item answered that was numbered highest.
- • Number of omissions (C2): the number of omissions was the number of items left blank prior to the last item answered. For PMA-SR, C2 + (20-B2) = C1 and for DAT-SR C2 + (50-B2) = C1.
Performance is measured by A3 indicator, which in DAT coincides with A1 whereas in PMA it also involves A2 for its calculation. B1 and B2 are speed indicators. C2 and C1 are used for measuring confidence, as they can differentiate whether an item is blank because of doubts in the correct answer or because of lack of time to answer it. The ratios of the number of correct answers and the number of items omitted to the number of items answered were used to infer the effectiveness of the strategy deployed ( Goldstein et al., 1990 ; Delgado and Prieto, 1996 ):
- • Number of correct answers/number of items answered (AR1).
- • Number of items omitted/number of items answered (CR2).
Data Analysis
In order to perform statistical analyses of data, those subjects whose protocols were incomplete or showed errors were removed from the analysis. First, the mean and standard deviation in the different scores was calculated (see Table 1 ), and the Kolmogorov–Smirnov test was used to assess the distribution of the scores. Determining the potential differences between groups in all variables was achieved through bifactorial intergroup 2 × 2 ANOVAs taking Gender and Ability as independent variables, and the scores obtained in PMA-SR and DAT-SR (absolute and ratio values) as dependent variables. Effect size was measured as partial eta-squared ( η p 2 ) and statistical significance was set at a confidence interval of 95%, with p < 0.05 as the accepted level of significance. All the analyses were performed using SPSS v.19 for Windows.
Mean, standard deviation, and F -values for the parameters describing dependent variables mathematical talent and gender, expressed as absolute values: PMA-SR and DAT-SR tests.
Mean, standard deviations, and F -values for the parameters describing dependent variables mathematical talent and gender, expressed as the ratio to the number of items answered: PMA-SR and DAT-SR.
Absolute Values
CPs scored significantly higher than NCPs in all the performance indicators in both tests: more correct answers (A1) [ F (1,323) = 77.60, p = 0.000, η p 2 = 0.194 in PMA-SR; F (1,323) = 127.47, p = 0.000, η p 2 = 0.283 in DAT-SR]; fewer incorrect answers (A2) [ F (1,323) = 46.60, p = 0.000, η p 2 = 0.126 in PMA-SR; F (1,323) = 116.95, p = 0.000, η p 2 = 0.226 in DAT-SR]; and a higher score (A3) [ F (1,323) = 58.41, p = 0.000, η p 2 = 0.153 in PMA-SR; F (1,323) = 127.47, p = 0.000, η p 2 = 0.283 in DAT-SR].
Gender had a significant effect on two of the performance indicators in PMA-SR, with males answering more items correctly (A1) [ F (1,323) = 5.86, p = 0.016, η p 2 = 0.016] and scoring higher [ F (1,323) = 5.84, p = 0.016, η p 2 = 0.018]. The differences in the number of incorrect responses (A2) were not statistically significant, however. Gender was not observed to prominently affect any of the performance indicators in DAT-SR. Nor was any significant interaction between the independent variables identified in any of the performance indicators in either test.
The CPs scored consistently higher in the speed indicators than the NCPs: more items attempted (B1) [ F (1,323) = 12.29, p = 0.001, η p 2 = 0.037 in PMA-SR; F (1,323) = 8.42, p = 0.004, η p 2 = 0.025 in DAT-SR] and a larger number of last items answered (B2) [ F (1,323) = 12.55, p = 0.000, η p 2 = 0.037 in PMA-SR; F (1,323) = 10.04, p = 0.002, η p 2 = 0.030 in DAT-SR].
In the PMA-SR test male subjects earned higher speed indicator scores, answered more items (B1) [ F (1,323) = 7.36, p = 0.007, η p 2 = 0.022] and completed more of the test by number of items answered (B2) than females [ F (1,323) = 4.26, p = 0.040, η p 2 = 0.013]. In contrast, gender had no significant effect on the DAT-SR test speed indicators, nor was any inter-variable interaction observed for speed in either of the two tests.
Problem-solving capacity exerted no prominent effect on the number of items omitted (C2) in either test, although talented complex problem-solvers left significantly fewer items blank (C1) [ F (1,323) = 12.29, p = 0.001, η p 2 = 0.037 in PMA-SR; F (1,323) = 8.42, p = 0.004, η p 2 = 0.025 in DAT-SR].
Although no differences were observed between the sexes in the total number of items left blank in the DAT-SR test, obvious differences were recorded in the number omitted (C2) [ F (1,323) = 6.85, p = 0.009, η p 2 = 0.021].
The gender differences in the number of speed-related blank items found in PMA-SR were not observed in connection with omissions. In this test the mean number of omissions was less than half an item, an indication that subjects only exceptionally failed to answer due to uncertainty. As in the other indicators, no inter-variable interaction was observed in omissions.
CPs exhibited significantly higher AR1 scores than NCPs in both tests, denoting a higher percentage of correct answers and fewer errors [ F (1,323) = 78.61, p = 0.000, η p 2 = 0.196 in PMA-SR; F (1,323) = 128.24, p = 0.000, η p 2 = 0.284, in DAT-SR]. Only minor differences were observed between the two groups in the number of items omitted, however, confirming the effectiveness of the non-omission strategy.
Males’ statistically significant higher absolute performance in terms of number of correct answers, scores and number of items answered in the PMA-SR test was absent in the AR1 findings. In other words, the differences between the sexes in the fraction of correct answers relative to the number of items answered were not significant.
In DAT-SR, as in the case of the absolute values which showed no differences in performance by sex, the AR1 ratio revealed the absence of significance between males’ and females’ likelihood of responding correctly to the items answered. In contrast, a significantly higher ratio of items omitted to items answered was observed for females (CR2) [ F (1,323) = 5.11, p = 0.024, η p 2 = 0.016].
This study used two spatial tests, PMA-SR and DAT-SR, to analyze the effect of gender and the ability to solve complex mathematical problems on performance. Gender (male/female) and mathematical ability (complex problem solvers/non-solvers) were the independent variables, while the performance indicators were score, number of correct and incorrect answers, number of items attempted, number left blank, number omitted and the last item answered, along with the ratios of the number of correct answers and the number of omissions to the total number of items answered. The study’s four major contributions to the effect of gender and mathematical talent on spatial aptitudes are highlighted below.
Performance Was Higher Among Students With Complex Mathematical Problem-Solving Talent Than Among Their Less Talented Peers
CP students performed better and faster than NCPs in both tests administered here. The former were found to score significantly better than the latter in both tests: making fewer mistakes, leaving fewer items blank, answering more items, and exhibiting a higher success rate per item answered. The present findings therefore corroborate the positive relationship between mathematical talent and visual ability reported earlier ( Rivera, 2011 ; Ramírez-Uclés et al., 2013 ; Rabab’h and Veloo, 2015 ; Ramírez and Flores, 2017 ), for the CP students in the sample implemented efficient test strategies, answering rapidly and omitting very few items.
No Interaction Was Identified Between Ability to Solve Complex Problems and Gender
Although gender differences have been frequently and separately reported in studies of mathematical performance and visual skills, no interaction was observed in any of the indicators analyzed here. When explored together, the effect of one variable on the other was not determinant and the differences in mathematical ability were unrelated to the gender differences found in the tests. Nor did gender determine the differences observed in mathematical ability. Unlike other studies, the research conducted here was unable to confirm that differences between the sexes revealed by spatial tests concur with differences in complex problem-solving abilities ( Olszewski-Kubilius and Turner, 2002 ). Nor was evidence found that such differences impact mathematical performance ( Ganley and Vasilyeva, 2011 ). Although differences between the sexes in some indicators were apparently narrower in the CP group than in the sample as a whole, they were not statistically significant.
None of the Indicators Denoted Significant Gender Differences in Both Tests
The inference drawn from the data, according to which none of the indicators denoted gender differences in both tests, is that the differences between the sexes in the performance factors were related to characteristics specific to each test. In other words, this study failed to find males more visually skilled, faster or more confident, for the differences in men’s and women’s scores were not observed consistently across the instruments and assessment criteria applied ( Stumpf and Eliot, 1995 ; Gibbs, 2010 ). That boys scored significantly higher than girls in the PMA test while sex had no prominent effect of on the DAT test scores would seem to confirm that gender differences are better substantiated in mental rotation tests than in other spatial tests, as often described elsewhere (e.g., Voyer et al., 1995 ; Moè, 2009 ; Xu et al., 2016 ).
In this study, the performance differences observed in the PMA-SR test were speed-related, with males answering more items and completing more of the test, although at a success rate no higher than the females’ in any of the items. In this test, boys implemented a better strategy because it was faster, whereas they did not outperform the females in terms of success per item or number of omissions. Therefore, the strategy of answering more items per unit of time yields more correct responses per unit of time, as reported by other authors for mental rotation tests ( Delgado and Prieto, 1996 ). The fact that only 9% of the subjects completed the PMA-SR test compared to 70% who completed the DAT-SR test attests to the need to answer more speedily to complete the former.
No differences between the sexes were observed in the speed or effectiveness indicators for DAT-SR. Differences were observed in that test with respect to omissions, with females more willing to leave an item blank when they were unsure of the answer. That finding was not consistent with results reported for an abridged version of the DAT-SR test, which revealed significant gender differences in the number of correct answers and items answered, but not in the absolute number of omissions or the ratio of omissions to the items answered ( Delgado and Prieto, 1996 ). The characteristics of the two studies differed, however. Firstly, the earlier authors used an abridged version of DAT-SR (30 items) that was administered to two groups, one of which was allowed 12 and the other 25 min to complete the test. As that difference in timing spawned significant differences in the success rates relative to the items attempted, the effectiveness of the test was conditioned by that parameter. Secondly, in the present study the CPs performed better and faster, confirming that they differed significantly from the NCPs in respect of their mathematical skills. Similarly, 70% of the subjects in this research completed the full version of the DAT-SR (50 items in 20 min), compared to only 27.2% of the students in the earlier study who were given the same amount of time in items per minute.
Gender-related differences in strategy implemented varied depending on the test. In the PMA boys deployed faster strategies, whereas in the DAT test girls proved more reluctant to guess.
Differences Between Absolute Variables and Ratios
The findings for the CP group were the same whether expressed as the absolute value of the variables or the value relative to the number of items attempted. The absolute DAT test results were likewise unchanged in any of the indicators when ratioed to the number of items attempted. In PMA-SR in contrast, the differences observed between the sexes in the absolute number of correct answers were absent when expressed as a fraction of the number of items answered, as observed by earlier authors ( Goldstein et al., 1990 ; Stumpf, 1993 ). The strategy indicator ‘number of omissions’ yielded the same results in absolute and relative terms, a finding also consistent with other reports ( Delgado and Prieto, 1996 ). In light of such disparity, the use of variable ratios cannot be said to necessarily narrow the gender gap observed.
Implications and Limitations
Two limitations to this study are sample size and the smaller proportion of women. In relation to the sample, the results obtained are specific to the Spanish students who participated in the study, using the ability to solve complex mathematical problems as an indicator of mathematical ability, and the results obtained in PMA and DAT test as an indicator of spatial ability. Further generalization of the results of this study about gender differences in mathematical performance and visualization should take this limitation into account, as well as the heterogeneity of students with mathematical talent ( Pitta-Pantazi and Christou, 2009 ). Another limitation stems from the smaller proportion of women in the sample selected, derived from their lower presence in the group of students selected to solve complex mathematical problems. Again, the results of this study should be interpreted under this limitation, which can itself be considered an indicator of sexes differences as found in certain contexts about mathematical abilities ( Hyde et al., 1990 ; Hyde, 2014 ). In this sense, we consider that the assumption that females are not as capable in solving complex mathematical problems or spatial visualization tasks compared to males is wide-spread and, moreover, has often the character of a prejudice that may condition girls to not participate in some mathematical programs. It is necessary to investigate the specific factors that motivate these differences and not consider them as a “simple” effect of gender that may influence decisions in educational and social fields.
The inequalities between the CP and control groups were consistent with previous reports ( Else-Quest et al., 2013 ; Hyde, 2014 ). This line of research would also benefit from a comparison to the results for other spatial tests and performance indicators. The present findings are nonetheless deemed to have significant implications, particularly for identifying gifted students or the direction adopted in future assessments of mathematical performance and visual ability. Affective factors associated with performance, speed or self-confidence have been shown to play different roles. In other words, the effect of greater self-confidence, greater speed or greater reluctance to guess on visual capacity might differ depending on the test. For instance, two subjects who work at different speeds might earn different scores in PMA-SR but the same in DAT-SR. By the same token, if one subject is more reluctant to guess than another, the two might earn the same scores in PMA-SR, but perform differently in DAT-SR. Just as the use of several instruments is recommended to identify gifted students ( Pitta-Pantazi and Christou, 2009 ), the present authors believe a number of instruments should be deployed to assess visual ability and how they are impacted by other factors.
Although some of the test scores attest to differences between the sexes, an analysis of the cognitive aspects associated with such differences is believed to be in order. Despite the dependence of the reluctance to guess on personality factors, the parameter of greatest relevance may be the time invested in mentally rotating objects rather than the speed in answering or the decision to answer an item.
Data Availability Statement
Ethics statement.
The studies involving human participants were reviewed and approved by the Bioethics Committee of the University of Granada. Written informed consent to participate in this study was provided by the participants’ legal guardian/next of kin.
Author Contributions
IR-U performed the overall planning and design of the study, the bibliographical revision, and the methodology and statistical analyses in the study. RR-U performed the assessment of the subjects, wrote the theoretical background, and derived the conclusions according to the results in the study.
Conflict of Interest
The authors declare that the research was conducted in the absence of any commercial or financial relationships that could be construed as a potential conflict of interest.
- Alansari B. M., DerÊgowski J. B., McGeorge P. (2008). Sex differences in spatial visualization of Kuwaiti school children. Soc. Behav. Pers. 36 811–824. 10.2224/sbp.2008.36.6.811 [ CrossRef ] [ Google Scholar ]
- Arcavi A. (2003). The role of visual representations in the learning of mathematics. Educ. Stud. Math. 52 215–241. [ Google Scholar ]
- Battista M. T. (1990). Spatial visualization and gender differences in high school geometry. J. Res. Math. Educ. 21 47–60. [ Google Scholar ]
- Benbow C. P., Stanley J. C. (1996). Inequity in equity: how “equity’ can lead to inequity for high potential students. Psychol. Public Policy Law 2 249–292. 10.1037/1076-8971.2.2.249 [ CrossRef ] [ Google Scholar ]
- Bennett G. K., Seashore H. G., Wesman A. G. (2000). Test de Aptitudes Diferenciales (DAT-5). Madrid: TEA Ediciones. [ Google Scholar ]
- Bishop A. J. (1980). Spatial abilities and mathematics education: a review. Educ. Stud. Math. 11 257–269. 10.1007/bf00697739 [ CrossRef ] [ Google Scholar ]
- Blum G., Auné S., Attorresi H. F. (2015). Desempeño de varones y mujeres en relación con el razonamiento visuoespacial. Influencia de la velocidad de respuesta, la educación y la presencia de rotación en los items. Rev. Mex. Psicol. 32 16–26. [ Google Scholar ]
- Campos A. (2014). Gender differences in imagery. Pers. Individ. Differ. 59 107–111. 10.1016/j.paid.2013.12.010 [ CrossRef ] [ Google Scholar ]
- Clements D. H., Battista M. T. (1992). “ Geometry and spatial reasoning ,” in Handbook of Research on Mathematics Teaching and Learning , ed. Grouws D. A. (New York, NY: MacMillan; ), 420–464. [ Google Scholar ]
- Contreras M. J., Rubio V. J., Peña D., Colom R., Santacreu J. (2007). Sex differences in dynamic spatial ability: the unsolved question of performance factors. Mem. Cogn. 35 297–303. 10.3758/bf03193450 [ PubMed ] [ CrossRef ] [ Google Scholar ]
- Cooke-Simpson A., Voyer D. (2007). Confidence and gender differences on the mental rotations test. Learn. Individ. Diff. 17 181–186. 10.1016/j.lindif.2007.03.009 [ CrossRef ] [ Google Scholar ]
- Delgado A. R., Prieto G. (1996). Sex differences in visuospatial ability: do performance factors play such an important role? Mem. Cogn. 24 504–510. 10.3758/bf03200938 [ PubMed ] [ CrossRef ] [ Google Scholar ]
- Else-Quest N. M., Hyde J. S., Linn M. C. (2010). Cross-national patterns of gender differences in mathematics: a meta-analysis. Psychol. Bull. 136 103–127. 10.1037/a0018053 [ PubMed ] [ CrossRef ] [ Google Scholar ]
- Else-Quest N. M., Mineo C. C., Higgins A. (2013). Math and science attitudes and achievement at the intersection of gender and ethnicity. Psychol. Women Q. 37 293–309. 10.1177/0361684313480694 [ CrossRef ] [ Google Scholar ]
- Feingold A. (1988). Cognitive gender differences are disappearing. Am. Psychol. 43 95–103. 10.1037/0003-066x.43.2.95 [ CrossRef ] [ Google Scholar ]
- Ganley C. M., Vasilyeva M. (2011). Sex differences in the relation between math performance, spatial skills, and attitudes. J. Appl. Dev. Psychol. 32 235–242. 10.1016/j.appdev.2011.04.001 [ CrossRef ] [ Google Scholar ]
- Geiser C., Lehmann W., Eid M. (2006). Separating “rotators” from “nonrotators” in the mental rotations test: a multigroup latent class analysis. Multivariate Behav. Res. 41 261–293. 10.1207/s15327906mbr4103-2 [ PubMed ] [ CrossRef ] [ Google Scholar ]
- Geiser C., Lehmann W., Eid M. (2008). A note on sex differences in mental rotation in different age groups. Intelligence 36 556–563. 10.1016/j.intell.2007.12.003 [ CrossRef ] [ Google Scholar ]
- Gibbs B. G. (2010). Reversing fortunes or content change? Gender gaps in math-related skill throughout childhood. Soc. Sci. Res. 39 540–569. 10.1016/j.ssresearch.2010.02.005 [ CrossRef ] [ Google Scholar ]
- Goldstein D., Haldane D., Mitchell C. (1990). Sex differences in visual-spatial ability: the role of performance factors. Mem. Cogn. 18 546–550. 10.3758/bf03198487 [ PubMed ] [ CrossRef ] [ Google Scholar ]
- Hall E. G. (1979). Sex differences in IQ development for intellectually gifted students. Roeper Rev. 2 25–28. 10.1080/02783198009552457 [ CrossRef ] [ Google Scholar ]
- Halpern D. E. (2000). Sex Differences in Cognitive Abilities , 3rd Edn, Hillsdale, NJ: Erlbaum. [ Google Scholar ]
- Halpern D. F., Benbow C. G., Geary R. C., Gur Shibley J. S., Gernsbacher M. A. (2007). The science of sex differences in science and mathematics. Psychol. Sci. Public Interest 8 1–51. [ PMC free article ] [ PubMed ] [ Google Scholar ]
- Hartlage L. C. (1970). Sex-linked inheritance of spatial ability. Percept. Mot. Skills 31 610–610. 10.2466/pms.1970.31.2.610 [ PubMed ] [ CrossRef ] [ Google Scholar ]
- Hedges L. V., Nowell A. (1995). Sex differences in mental test scores, variability, and numbers of high-scoring individuals. Science 269 41–45. 10.1126/science.7604277 [ PubMed ] [ CrossRef ] [ Google Scholar ]
- Hirnstein M., Bayer U., Hausmann M. (2009). Sex-specific response strategies in mental rotation. Learn. Individ. Differ. 19 225–228. 10.1016/j.lindif.2008.11.006 [ CrossRef ] [ Google Scholar ]
- Hong E., Aqui Y. (2004). Cognitive and motivational characteristics of adolescents gifted in mathematics: comparisons among students with different types of giftedness. Gift. Child Q. 48 191–201. 10.1177/001698620404800304 [ CrossRef ] [ Google Scholar ]
- Hyde J. S. (2014). Gender similarities and differences. Annu. Rev. Psychol. 65 373–398. [ PubMed ] [ Google Scholar ]
- Hyde J. S., Fennema E., Lamon S. (1990). Gender differences in mathematics performance: a meta-analysis. Psychol. Bull. 107 139–155. 10.1037/0033-2909.107.2.139 [ PubMed ] [ CrossRef ] [ Google Scholar ]
- Hyde J. S., Lindberg S. M., Linn M. C., Ellis A., Williams C. (2008). Gender similarities characterize math performance. Science 3 494–495. 10.1126/science.1160364 [ PubMed ] [ CrossRef ] [ Google Scholar ]
- Kail R., Stevenson M. R., Black K. N. (1984). Absence of a sex difference in algorithms for spatial problem-solving. Intelligence 8 37–46. 10.1016/0160-2896(84)90005-9 [ CrossRef ] [ Google Scholar ]
- Kaufman S. B. (2007). Sex differences in mental rotation and spatial visualization ability: can they be accounted for by differences in working memory capacity? Intelligence 35 211–223. 10.1016/j.intell.2006.07.009 [ CrossRef ] [ Google Scholar ]
- Krutetskii V. A. (1976). The Psychology of Mathematical Abilities in Schoolchildren. Chicago, IL: University of Chicago Press. [ Google Scholar ]
- Lean G. Y., Clements M. A. (1981). Spatial ability, visual imagery, and mathematical performance. Educ. Stud. Math. 12 267–299. 10.1007/bf00311060 [ CrossRef ] [ Google Scholar ]
- Lindberg S. M., Hyde J. S., Petersen J., Linn M. C. (2010). New trends in gender and mathematics performance: a meta-analysis. Psychol. Bull. 136 1123–1135. 10.1037/a0021276 [ PMC free article ] [ PubMed ] [ CrossRef ] [ Google Scholar ]
- Linn M. C., Petersen A. C. (1985). Emergence and characterization of sex differences in spatial ability: a meta-analysis. Child Dev. 56 1479–1498. [ PubMed ] [ Google Scholar ]
- Loring-Meier S., Halpern D. F. (1999). Sex differences in visuospatial working memory: components of cognitive processing. Psychonomic Bull. Rev. 6 464–471. 10.3758/BF03210836 [ PubMed ] [ CrossRef ] [ Google Scholar ]
- Low R., Over R. (1993). Gender differences in solution of algebraic word problems containing irrelevant information. J. Educ. Psychol. 85 331–339. 10.1037/0022-0663.85.2.331 [ CrossRef ] [ Google Scholar ]
- Maccoby E. E., Jacklin C. N. (1974). The Psychology of Sex Differences. Stanford, CA: Stanford University Press. [ Google Scholar ]
- Maeda Y., Yoon S. Y. (2016). Are gender differences in spatial ability real or an artifact? Evaluation of measurement invariance on the revised PSVT: R. J. Psychoeduc. Assess. 34 397–403. 10.1177/0734282915609843 [ CrossRef ] [ Google Scholar ]
- Masters M. S. (1998). The gender difference on the mental rotations test is not due to performance factors. Mem. Cogn. 26 444–448. 10.3758/bf03201154 [ PubMed ] [ CrossRef ] [ Google Scholar ]
- Moè A. (2009). Are males always better than females in mental rotation? Exploring a gender belief explanation. Learn. Individ. Differ. 19 21–27. 10.1016/j.lindif.2008.02.002 [ CrossRef ] [ Google Scholar ]
- Olszewski-Kubilius P., Turner D. (2002). Gender differences among elementary school-aged gifted students in achievement, perceptions of ability, and subject preference. J. Educ. Gift. 25 233–268. 10.1177/016235320202500303 [ CrossRef ] [ Google Scholar ]
- Parsons J. E., Adler T. F., Kaczala C. M. (1982). Socialization of achievement attitudes and beliefs: parental influences. Child Dev. 53 310–321. [ Google Scholar ]
- Peters M. (2005). Sex differences and the factor of time in solving Vandenberg and Kuse mental rotation problems. Brain Cogn. 57 176–184. 10.1016/j.bandc.2004.08.052 [ PubMed ] [ CrossRef ] [ Google Scholar ]
- Pitta-Pantazi D., Christou C. (2009). “ Psychological aspect: identification of giftedness in earlier ages ,” in Proceedings of the 33rd Conference of the International Group for the Psychology of Mathematics Education , eds Tzekaki M., Kaldrimidou M., Sakonidis Y. H. (Thessaloniki, GR: PME; ), 191–194. [ Google Scholar ]
- Presmeg N. (1986). Visualisation and mathematical giftedness. Educ. Stud. Math. 17 297–311. 10.1007/bf00305075 [ CrossRef ] [ Google Scholar ]
- Rabab’h B., Veloo A. (2015). Spatial visualization as mediating between mathematics learning strategy and mathematics achievement among 8th grade students. Int. Educ. Stud. 8 1–11. [ Google Scholar ]
- Ramírez R., Flores P. (2017). Habilidades de visualización de estudiantes con talento matemático: comparativa entre los test psicométricos y las habilidades de visualización manifestadas en tareas geométricas. Enseñanza de las Ciencias 35 179–196. [ Google Scholar ]
- Ramírez-Uclés R., Ramírez-Uclés I., Flores P., Castro E. (2013). Analysis of spatial visualization and intellectual capabilities in mathematically gifted students. Rev. Mex. Psicol. 30 24–31. [ Google Scholar ]
- Reis S. M., Park S. (2001). Gender differences in high-achieving students in math and science. J. Educ. Gift. 25 52–73. 10.1371/journal.pone.0222357 [ PMC free article ] [ PubMed ] [ CrossRef ] [ Google Scholar ]
- Rivera F. D. (2011). Towards a Visually-Oriented School Mathematics Curriculum. New York, NY: Springer. [ Google Scholar ]
- Robert M., Chevrier E. (2003). Does men’s advantage in mental rotation persist when real three-dimensional objects are either felt or seen? Mem. Cogn. 31 1136–1145. 10.3758/BF03196134 [ PubMed ] [ CrossRef ] [ Google Scholar ]
- Steinmayr R., Spinath B. (2008). Sex differences in school achievement: what are the roles of personality and achievement motivation? Eur. J. Pers. 22 185–209. 10.1002/per.676 [ CrossRef ] [ Google Scholar ]
- Stericker A., LeVesconte S. (1982). Effect of brief training on sex-related differences in visual-spatial skill. J. Pers. Soc. Psychol. 43 1018–1029. 10.1037/0022-3514.43.5.1018 [ PubMed ] [ CrossRef ] [ Google Scholar ]
- Strand S., Deary I. J., Smith P. (2006). Sex differences in cognitive abilities test scores: a UK national picture. Br. J. Educ. Psychol. 76 463–480. 10.1348/000709905X50906 [ PubMed ] [ CrossRef ] [ Google Scholar ]
- Stumpf H. (1993). Performance factors and gender-related differences in spatial ability: another assessment. Mem. Cogn. 21 828–836. 10.3758/bf03202750 [ PubMed ] [ CrossRef ] [ Google Scholar ]
- Stumpf H., Eliot J. (1995). Gender-related differences in spatial ability and the k factor of general spatial ability in a population of academically talented students. Pers. Individ. Differ. 19 33–45. 10.1016/0191-8869(95)00029-6 [ CrossRef ] [ Google Scholar ]
- Thurstone L. L., Thurstone T. G. (1976). P.M.A. Aptitudes Mentales Primarias. Madrid: TEA. [ Google Scholar ]
- Voyer D. (2011). Time limits and gender differences on paper-and-pencil tests of mental rotation: a meta-analysis. Psychonom. Bull. Rev. 18 267–277. 10.3758/s13423-010-0042-0 [ PubMed ] [ CrossRef ] [ Google Scholar ]
- Voyer D., Rodgers M. A., McCormick P. A. (2004). Timing conditions and the magnitude of gender differences on the mental rotations test. Mem. Cogn. 32 72–82. 10.3758/bf03195821 [ PubMed ] [ CrossRef ] [ Google Scholar ]
- Voyer D., Saunders K. A. (2004). Gender differences on the mental rotations test: a factor analysis. Acta Psychol. 117 79–94. 10.1016/j.actpsy.2004.05.003 [ PubMed ] [ CrossRef ] [ Google Scholar ]
- Voyer D., Voyer S., Bryden M. P. (1995). Magnitude of sex differences in spatial abilities: a meta-analysis and consideration of critical variables. Psychol. Bull. 117 250–270. 10.1037/0033-2909.117.2.250 [ PubMed ] [ CrossRef ] [ Google Scholar ]
- Voyer D., Voyer S. D. (2014). Gender differences in scholastic achievement: a meta-analysis. Psychol. Bull. 140 1174–1204. 10.1037/a0036620 [ PubMed ] [ CrossRef ] [ Google Scholar ]
- Wach F. S., Spengler M., Gottschling J., Spinath F. M. (2015). Sex differences in secondary school achievement: the contribution of self-perceived abilities and fear of failure. Learn. Instruct. 36 104–112. 10.1016/j.learninstruc.2015.01.005 [ CrossRef ] [ Google Scholar ]
- Xu X., Kim E. S., Lewis J. E. (2016). Sex difference in spatial ability for college students and exploration of measurement invariance. Learn. Individ. Differ. 45 176–184. 10.1016/j.lindif.2015.11.015 [ CrossRef ] [ Google Scholar ]
- Yoon S. Y., Mann E. L. (2017). Exploring the spatial ability of undergraduate students: association with gender, STEM majors, and gifted program membership. Gift. Child Q. 61 313–327. 10.1177/0016986217722614 [ CrossRef ] [ Google Scholar ]
Advertisement
How Partner Gender Influences Female Students’ Problem Solving in Physics Education
- Published: 23 November 2006
- Volume 15 , pages 331–343, ( 2006 )
Cite this article
- N. Ding 1 &
- E. Harskamp 1
748 Accesses
18 Citations
1 Altmetric
Explore all metrics
Research has shown that female students cannot profit as much as male students can from cooperative learning in physics, especially in mixed-gender dyads. This study has explored the influence of partner gender on female students’ learning achievement, interaction and the problem-solving process during cooperative learning. In Shanghai, a total of 50 students (26 females and 24 males), drawn from two classes of a high school, took part in the study. Students were randomly paired, and there were three research groups: mixed-gender dyads (MG), female–female dyads (FF) and male–male dyads (MM). Analysis of students’ pre- and post-test performances revealed that female students in the single-gender condition solved physics problems more effectively than did those in the mixed-gender condition, while the same was not the case for male students. We further explored the differences between female and male communication styles, and content among the three research groups. It showed that the females’ interaction content and problem-solving processes were more sensitive to partner gender than were those for males. This might explain why mixed-gender cooperation in physics disadvantages females in high schools.
This is a preview of subscription content, log in via an institution to check access.
Access this article
Price includes VAT (Russian Federation)
Instant access to the full article PDF.
Rent this article via DeepDyve
Institutional subscriptions
Similar content being viewed by others
Draw Your Physics Homework?
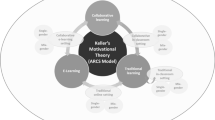
The impact of e-learning, gender-groupings and learning pedagogies in biology undergraduate female and male students’ attitudes and achievement
Study on Creative Learning Strategies of Gender Grouping in Collaborative Learning of Graduation Project
Bales R. F. (1950) Interaction Process Analysis: A Method for the Study of Small Groups, Reading, MA: Addison-Wesley
Google Scholar
Bales R. F. (1999) Social Interaction Systems: Theory and Measurement, New Brunswick: Transaction
Barbieri M. S., Light P. H. (1992) Interaction, gender, and performance on a computer-based problem solving task. Learning and Instruction 2: 199–213
Article Google Scholar
Cohen E. (1994) Restructuring the classroom: Situations for productive small groups. Review of Educational Research 64(1): 1–35
Ding N., Xu Y. R. (2005) “Giving students hints”—An investigation of improving students’ problem-solving skills in high school science learning. Asia-Pacific Forum on Science Learning 6(2)
Erkens, G. (1998). Multiple Episode Protocol Analysis (MEPA 3.0) , Internal publication. Department of Educational Sciences, Utrecht University, The Netherlands.
Fetler M. (1985) Sex differences on the California statewide assessment of computer literacy. Sex Roles 13 (3/4): 181–191
Hogan D., Tudge J. (1999) Implications of Vygotsky’s theory for peer learning. In O’Donnell A., King A. (Eds.), Cognitive Perspectives on Peer Learning. Mahwah, NJ: Lawrence Erlbaum Associates
Howe C., Tolmie A., Greer K., Mackenzie M.(1995) Peer collaboration and conceptual growth in physics: Task influences on children’s understanding of heating and cooling. Cognition and Instruction 13(4): 483–503
Hyde J. S., Fennema E., Lamon. S. J. (1990) Gender differences in mathematics performance: A meta-analysis. Psychological Bulletin 107(2): 139–155
Johnson D. W., Johnson R. T. (1986) Computer-assisted cooperative learning. Educational Technology 26(1): 12–18
Lakoff R. (1973) Language and woman’s place. Language in Society 2: 45–80
Lay M. M. (1992) The androgynous collaborator: The impact of gender studies on collaboration. In Forman J. (ed.), New Visions of Collaborative Writing, Portsmouth, NH: Boynton/Cook, pp. 82–104
Lehtinen, E. (2003). Computer-supported collaborative learning: An approach to powerful learning environments. In Powerful Learning Environments: Unravelling Basic Components and Dimensions , Pergamon, pp. 35–53
Li Q. (2002) Gender and computer-mediated communication: An exploration of elementary students’ mathematics and science learning. Journal of computers in mathematics and science teaching 21(4): 341–359
Light P., Littleton K., Bale S., Joiner R., Messer D. (2000) Gender and social comparison effects in computer-based problem solving. Learning and Instruction 10: 483–496
Margrett J. A., Marsiske M. (2002) Gender differences in older adults’ everyday cognitive collaboration. International Journal of Behavioral Development. 26(1): 45–59
Mercer N. (1996) the quality of talk in children’s collaborative activity in the classroom. Learning and Instruction 6: 359–377
Orenstein P. (1994) School Females. Doubleday: NY
Pol H., Harskamp E., Suhre C. (2005) The solving of physics problems: Computer assisted instruction. International Journal of Science Education 27: 451–469
Schoenfeld A. H. (1992) Learning to think mathematically: Problem solving, metacognition, and sense making in mathematics. In Grouws D. A. (Ed.), Handbook of Research on Mathematics Teaching and Learning. NY: Macmillan, pp. 334–367
Schwartz D. L. (1995) The emergence of abstract representations in dyad problem solving. Journal of the Learning Sciences 4(3): 321–354
Sharan S., Shachar H. (1988) Language and learning in the cooperative classroom. New York: Springer
Sherin B. L. (2001) How students understand physics equations. Cognition and Instruction 19(4): 479–541
Siann G., Macleod H. (1986) Computers and children of primary school age: issues and questions. British Journal of Educational Technology 17: 199–44
Siann G., Durndell A., Macleod H., Glissov P. (1988) Stereotyping in relation to gender gap in participation in computing. Educational Research 30: 98–103
Speck, B. W. (2003). Fostering collaboration among students in problem-based learning. In New Directions for Teaching and Learning , Wiley Periodicals, Inc. p. 59, 95
Sutherland L. (2002) Developing problem solving expertise: The impact of instruction in a question analysis strategy. Learning and Instruction 12: 155–187
Teasley S. (1995) The role of talk in children’s peer collaboration. Developmental Psychology 3(2): 207–220
Underwood G., Jindal N., Underwood J. D. M. (1994) Gender differences and effects of co-operation in a computer-based language task. Educational Research 36: 63–74
Webb, N. M. (1984). Sex Differences in Interaction and Achievement in Cooperative Small Groups. Journal of Educational Psychology 36(1): 33–44.
Download references
Author information
Authors and affiliations.
Faculty of Behavioral and Social Sciences, University of Groningen, PO Box 1286, 9701 BG, Groningen, The Netherlands
N. Ding & E. Harskamp
You can also search for this author in PubMed Google Scholar
Corresponding author
Correspondence to N. Ding .
Rights and permissions
Reprints and permissions
About this article
Ding, N., Harskamp, E. How Partner Gender Influences Female Students’ Problem Solving in Physics Education. J Sci Educ Technol 15 , 331–343 (2006). https://doi.org/10.1007/s10956-006-9021-7
Download citation
Published : 23 November 2006
Issue Date : December 2006
DOI : https://doi.org/10.1007/s10956-006-9021-7
Share this article
Anyone you share the following link with will be able to read this content:
Sorry, a shareable link is not currently available for this article.
Provided by the Springer Nature SharedIt content-sharing initiative
- cooperative learning
- interaction
- problem solving
- physics education.
- Find a journal
- Publish with us
- Track your research

- SUGGESTED TOPICS
- The Magazine
- Newsletters
- Managing Yourself
- Managing Teams
- Work-life Balance
- The Big Idea
- Data & Visuals
- Reading Lists
- Case Selections
- HBR Learning
- Topic Feeds
- Account Settings
- Email Preferences
Teams Solve Problems Faster When They’re More Cognitively Diverse
- Alison Reynolds
- David Lewis

Find people who disagree with you and cherish them.
Looking at the executive teams we work with as consultants and those we teach in the classroom, increased diversity of gender, ethnicity, and age is apparent. Over recent decades the rightful endeavor to achieve a more representative workforce has had an impact. Of course, there is a ways to go, but progress has been made.
- AR Alison Reynolds is a member of faculty at the UK’s Ashridge Business School where she works with executive groups in the field of leadership development, strategy execution and organization development. She has previously worked in the public sector and management consulting, and is an advisor to a number of small businesses and charities.
- DL David Lewis is Director of London Business School’s Senior Executive Programme and teaches on strategy execution and leading in uncertainty. He is a consultant and works with global corporations, advising and coaching board teams. He is co-founder of a research company focusing on developing tools to enhance individual, team and organization performance through better interaction.
Partner Center

IMAGES
VIDEO
COMMENTS
This work examines gender gaps in collaborative problem solving in the teenage years in the 2015 PISA. Girls outperform boys in collaborative problem solving in all countries in the study. At the country level, the size of the gender gap depends on societal-level gender equality, although associations depend on how societal-level gender equality is operationalized.
5 Testosterone is a kind of male sex hormone. 6 Estradiol is a type of estrogen, which is a kind of female sex hormone. their male peers) and females with low levels of estradiol (compared to their female peers) would have higher level of spatial abilities. This theory needs strict confirmation from other studies.
Of particular interest in this analysis was whether a gender difference in complex problem solving would be seen among high school and college students, as was found in Hyde's 1990 meta-analysis. Our results showed that there was a small gender difference favoring high school males on tests that included problems at Levels 3 or 4 ( d = +0.16 ...
In high school, boys showed a slight edge in problem solving, possibly because they took more science classes that emphasized those skills. But boys and girls understood math concepts equally well, and any gender differences actually narrowed over the years, belying the notion of a fixed or biological differentiating factor.
These findings have been substantiated in experimental studies, where the gender identity of the instructor in online courses was manipulated, ... (Hofstra et al., 2020), as well as increased problem-solving (Hong and Page, 2004) and scientific discovery (Nielsen et al., 2018).
Introduction. Although the importance of visualization in mathematical problem solving has been highlighted in mathematics education (Clements and Battista, 1992; Arcavi, 2003), no consensus has yet been reached on its role in improving performance (Bishop, 1980; Lean and Clements, 1981).Traditional studies concluded that spatial awareness and the capacity to visualize abstract mathematical ...
We provide new empirical evidence on gender differences among 15-year-old students in the ability to engage effectively in goal-directed collaboration and consider whether the between-country variation in the gender gap in collaborative problem solving is related to measures of societal-level gender equality.
Effective collaborative problem solving comprises cognitive dimensions, in which men tend to outperform women, and social dimensions in which women tend to outperform men. We extend research on between-country differences in gender gaps by considering collaborative problem solving and its association with two indicators of societal-level gender inequality. The first indicator reflects women's ...
Evidence for gender effects in problem-solving is heterogeneous, although there is some evidence that problem-solving performance varies between genders according to the problem domain (Walker et ...
Collaborative learning has been proved to be an effective learning method that can benefit group members more than individual learning. However, these benefits do not extend equally to boys and girls. Many studies have been conducted to explore the effects and features of different gender groups, but they have presented conflicting findings, which possibly resulted from variations in task type ...
A meta-analysis of studies looking at simulated employment decisions (N = 22,348) found little or no gender bias in female-dominated or gender-balanced fields, but a small-to-moderate pro-male bias among males in male-dominated fields (d = 0.3; Koch et al., 2015, Table 2).
Abstract. In this study, we investigated gender differences in problem solving as a function of problem context and expectations for success. Subjects were 90 women and 56 men from introductory ...
Prior studies have indicated that differences in mathematical performance rely on the kind of task students are asked to complete. These studies have found that females perform better in arithmetic and calculus, while males perform better in mathematical problem solving (Byrnes and Takahira, 1993). However, there have also been conflicting ...
School District 3 Comer Brook, Newfoundland, Canada. The relationship between gender and mathematical problem-solving among high ability students depends on the attributes of the problem solving questions. This was evident in the present study of 12-year-olds. The children were from predominately White families.
Strategies for integrating gender equity in teaching problem-solving. ... Mitchell & Martin, 2018). Studies have shown how gender stereotypes have affected the learning outcomes of learners, especially female learners, and how they have influenced their choices in STEM careers (Tsouroufli & Redai, 2021). Teachers need to avoid such situations ...
Browse 192 studies aiming to close gender gaps. How Partner Gender Influences Female Students' Problem Solving in Physics Education. ... While there is no clear empirical evidence as to whether female students' interaction style and problem-solving processes are influenced by their partner gender, it is assumed that the females' lack of ...
All of these studies confirm that the problem solving strategies are the most frequently used strategies in EFL reading learning. With respect to the online reading skills and the English proficiency, this study revealed that the female students gained better score than the male students, despite the fact that gender difference was not identified.
Examples include Canva, Piktochart, Adobe Spark and Easel.ly. Guide your students throughout this exercise, pointing out the goal (for example, to work towards the elimination of gender violence, discrimination or inequalities) and make the importance of generating creative solutions clear.
ABSTRACT. In this study, we investigated gender differences in problem solving as a function of problem context and expectations for success. Subjects were 90 women and 56 men from introductory psychology classes who were administered a set of mathematical problems varying in gender context under male-expectancy, female-expectancy, and neutral-expectancy conditions.
Previous studies have demonstrated gender differences in the emotional intelligence and empathy of nurses and medical students (Abe et al., 2018; ... We found no evidence of gender differences in problem-solving skills, diverging from previous studies (Yildirim et al., 2019). This result may be explained by different thought patterns and ...
Mathematical problem-solving and spatial visualization are areas in which performance has been shown to vary with sex. This article describes the impact of gender on spatial relations measured in 331 secondary school students (202 males, 129 females), 145 (105 males, 40 females) of whom had been selected to participate in a mathematical talent stimulation project after passing a complex ...
Research has shown that female students cannot profit as much as male students can from cooperative learning in physics, especially in mixed-gender dyads. This study has explored the influence of partner gender on female students' learning achievement, interaction and the problem-solving process during cooperative learning. In Shanghai, a total of 50 students (26 females and 24 males), drawn ...
Looking at the executive teams we work with as consultants and those we teach in the classroom, increased diversity of gender, ethnicity, and age is apparent.
In a separate study, Duffy et al. (1997) investigated the role of gender in twelve-year-old learners and found no difference in their mathematical problem-solving achievement.