Thank you for visiting nature.com. You are using a browser version with limited support for CSS. To obtain the best experience, we recommend you use a more up to date browser (or turn off compatibility mode in Internet Explorer). In the meantime, to ensure continued support, we are displaying the site without styles and JavaScript.
- View all journals
- Explore content
- About the journal
- Publish with us
- Sign up for alerts
- Open access
- Published: 11 March 2024

Long-term, sustainable solutions to radioactive waste management
- Kristina Kvashnina 1 , 2 ,
- Francis Claret 3 ,
- Nicolas Clavier 4 ,
- Tatiana G. Levitskaia 5 ,
- Haruko Wainwright 6 &
- Tiankai Yao 7
Scientific Reports volume 14 , Article number: 5907 ( 2024 ) Cite this article
3314 Accesses
3 Altmetric
Metrics details
- Geochemistry
- Nuclear waste
Nuclear power plays a pivotal role in ensuring a scalable, affordable, and reliable low-carbon electricity supply. Along with other low-carbon energy technologies, nuclear energy is essential for reducing our reliance on fossil fuels, addressing climate change and air pollution, and achieving a sustainable economy. Whilst significant progress has been made in reducing the volume of final radioactive waste, its management remains one of the most important challenges when considering the continued use and expansion of nuclear energy. This recently published collection highlights the latest technological and scientific advances aimed to improve the safe, long-term, and sustainable management of wastes produced from nuclear power generation.
Nuclear power is an ideal option for sustainable energy generation due to its long operating life and its ability to generate electricity with minimal greenhouse gas emissions. However, this form of energy generation produces radioactive waste that must be either securely stored and disposed or subjected to reprocessing. Long-term, sustainable solutions for radioactive waste management require a combination of technical expertise, regulatory oversight, and ongoing research to ensure the safe containment and ultimate disposal.
One approach is to establish deep geological repositories in stable geological formations. These repositories should be designed to isolate radioactive waste from the environment for thousands of years. In this context, Finkeldei, Huitinnen and colleagues investigated zirconia (ZrO 2 ) formed on spent nuclear fuel rod cladding and demonstrated its potential as an engineered barrier for immobilizing radionuclides 1 . They achieved this by structurally incorporating Eu 3+ and Cm 3+ into zirconia and analyzed the materials using complementary methods, including powder X-ray diffraction (PXRD), spectrum imaging analysis based on energy-dispersive X-ray spectroscopy in scanning transmission electron microscopy mode (STEM-EDXS), and luminescence spectroscopy. They showed that zirconia could be a suitable technical retention barrier for mobilized trivalent actinides in deep geological repositories.
Ceramic materials are also considered as leading candidate waste forms for the immobilization and geological disposal of long-lived actinides. In this collection, Corkhill et al. 2 investigated titanate ceramics with the pyrochlore structure using photoemission and X-ray absorption spectroscopy (XAS) and showed the possibility of chemical flexibility in terms of crystal-chemical design principles of pyrochlore structure. Additionally, Corkhill et al. 3 explored the mineral brannerite UTi 2 O 6 as a possible host phase for the immobilization of long-lived actinides, such as plutonium. Their investigation demonstrated that the ThTi 2 O 6 brannerite structure can incorporate a small fraction of U 6+ alongside a more significant inventory of U 5+ , achieved through charge compensation on the Th or Ti site. Blackburn et al. 4 conducted research on zirconolite as a candidate waste form material for immobilizing actinides from spent nuclear fuel. They considered indium as a neutron-absorbing additive to mitigate criticality in ceramic waste forms. The recorded K-edge spectroscopy data on In and Zr provide valuable information about the coordination environment and oxidation states of In and Zr, contributing to the performance testing of titanate waste form materials. Anionic clays like hydrotalcites are being explored, but their ability to serve as a durable repository for long-term actinide isolation is uncertain. Douglas and co-authors 5 showed that nanoscale hydrotalcite efficiently captured radionuclides, suggesting a potential rapid decontamination and long-term containment solution. Finally, spent nuclear fuel itself can be considered as a suitable wasteform, leading to its direct storage in geological repositories. In this context, Vinograd and co-workers 6 questioned the reactivity of Ln-doped UO 2 samples, as model compounds for SNF, towards oxidation in air. They showed that the partitioning of lanthanide cations between the original fluorite-type phase and the oxidized U 3 O 8 enhanced the resistivity to oxidation, thus contributing to the chemical durability of the ceramic.
Investing in fundamental research and the development of new technologies for waste treatment and disposal can lead to more efficient and cost-effective methods. Our collection features several articles on fundamental science, with a particular focus on investigating the electronic structure of actinide materials, which plays a significant role in radioactive waste management. Butorin and his co-authors 7 conducted research on americium oxide, an important component of the nuclear fuel cycle, using soft XAS and electronic structure calculations. Their study revealed that AmO 2 can be classified as a charge-transfer compound with a 5f. occupancy of 5.73 electrons, while Am 2 O 3 exhibits characteristics of a Mott–Hubbard system with a 5f occupancy of 6.05.
Investigating nuclear accidents is crucial for improving nuclear waste solutions because it enhances safety, reduces risks, strengthens regulations, drives technological advancements, and encourages international cooperation to address the challenges of nuclear waste management. Poliakova et al. 8 studied uranium oxides, which can disperse into the environment in various forms following different accidental scenarios. The team investigated the behavior of uranium oxide particles, which could be easily ingested by humans and animals in the vicinity of a contaminated area. Using several experimental methods, they observed structural changes in mixed uranium oxides, specifically UO 2, U 4 O 9 , U 3 O 8 and UO 3 , before and after exposure in simulated biological fluids (gastro-intestinal and lung). The most significant changes were observed in U 4 O 9 . This study heavily utilized XAS techniques at synchrotron sources, highlighting the crucial role of synchrotron radiation in nuclear waste research.
Understanding the chemical forms, defects, crystal structures, and other properties of radioactive materials is crucial before the construction of a final geological repository. While underpinning radionuclides speciation is a key asset to dive its mobility, reactive transport code allow to model their behavior on a long term scale. Tournassat et al. 9 have developed a user-friendly numerical reactive transport approach in order to tackle diffusion experiment in a smarter way. Reactive transport is a powerful tools but when uncertainty analysis have to be conducted, the computing cost could be a limiting factor. To overcome this issue surrogate model can be used. Turunen and Lipping 10 have built surrogate models using convolutional neural networks and used the meta model for a sensitivity analysis of the radionuclide transport models. In a separate work, Butorin and the team 11 investigated the effect of carbon content on the electronic structure of uranium carbides through a combination of XAS and density functional theory that takes 5f–5f Coulomb interaction U and spin–orbit coupling (DFT + U + SOC) into consideration. The results revealed an increase amount of 5f electrons in UC 2 as compared to UC, which will impact the thermodynamic properties and eventually on the carbide nuclear fuel performance.
Transmutation of radionuclides have been considered as one of the potential solutions for managing high-level radioactive waste. Sun et al. 12 proposed a new framework to transmute not only minor actinides but also long-lived fission products based on lead-cooled fact reactors. Using OpenMC, the optimal MA loading is evaluated based on the operation of the core, the neutron fux distribution, spectra, and k eff . They have shown the premise of transmuting mobile and key radionuclides for geological disposal such as Tc-99, with the higher transmutation rate than 15%. One more aspect, as proper decommissioning of nuclear facilities and reactors, followed by the management of resulting waste, is a critical component of long-term solutions. In this field, different strategies can be envisaged to lower both the volume and the radiotoxicity of the waste. The first one lies on the reprocessing of spent fuel in order to separate the different isotopes to offer targeted solutions for their storage. In this frame, Pilgrim et al. 13 proposed novel aminocarboxylate chelators providing highly efficient complexation of trivalent lanthanides and minor actinides. They particularly studied structural modifications of the ligands that increased their solubility without influencing their ability to complex lanthanides and minor actinides in solution.
Overall, we believe there are many key aspects which play a role in finding long-term solutions for radioactive waste. Proper classification of radioactive waste based on its characteristics and levels of radioactivity is essential. Strict safety standards and regulations are crucial to safeguard workers, the public, and the environment. Radioactive waste management often involves international cooperation, as some countries may not have the resources or expertise to manage their waste independently. Our collection reflects the international collaboration between scientists in different fields and in world-wide locations.
Opitz, L. et al. Investigations towards incorporation of Eu3+ and Cm3+ during ZrO2 crystallization in aqueous solution. Sci. Rep. 13 (1), 12276. https://doi.org/10.1038/s41598-023-39143-0 (2023).
Article ADS CAS PubMed PubMed Central Google Scholar
Sun, S.-K. et al. Crystal chemical design, synthesis and characterisation of U(IV)-dominant betafite phases for actinide immobilisation. Sci. Rep. 13 (1), 10328. https://doi.org/10.1038/s41598-023-36571-w (2023).
Dixon Wilkins, M. C. et al. A multimodal X-Ray spectroscopy investigation of uranium speciation in ThTi2O6 compounds with the brannerite structure. Sci. Rep. 13 (1), 12776. https://doi.org/10.1038/s41598-023-38912-1 (2023).
Blackburn, L. R. et al. Underpinning the use of indium as a neutron absorbing additive in zirconolite by X-ray absorption spectroscopy. Sci. Rep. 13 (1), 9329. https://doi.org/10.1038/s41598-023-34619-5 (2023).
Douglas, G. B. et al. Engineered mineralogical interfaces as radionuclide repositories. Sci. Rep. 13 (1), 2121. https://doi.org/10.1038/s41598-023-29171-1 (2023).
Vinograd, V. L., Bukaemskiy, A. A., Deissmann, G. & Modolo, G. Thermodynamic model of the oxidation of Ln-doped UO2. Sci. Rep. 13 (1), 17944. https://doi.org/10.1038/s41598-023-42616-x (2023).
Butorin, S. M. & Shuh, D. K. Chemical bonding in americium oxides probed by X-ray spectroscopy. Sci. Rep. 13 (1), 11607. https://doi.org/10.1038/s41598-023-38505-y (2023).
Poliakova, T. et al. Uranium oxides structural transformation in human body liquids. Sci. Rep. 13 (1), 4088. https://doi.org/10.1038/s41598-023-31059-z (2023).
Tournassat, C., Steefel, C. I., Fox, P. M. & Tinnacher, R. M. Resolving experimental biases in the interpretation of diffusion experiments with a user-friendly numerical reactive transport approach. Sci. Rep. 13 (1), 15029. https://doi.org/10.1038/s41598-023-42260-5 (2023).
Turunen, J. & Lipping, T. Feasibility of neural network metamodels for emulation and sensitivity analysis of radionuclide transport models. Sci. Rep. 13 (1), 6985. https://doi.org/10.1038/s41598-023-34089-9 (2023).
Butorin, S. M. et al. Effect of carbon content on electronic structure of uranium carbides. Sci. Rep. 13 (1), 20434. https://doi.org/10.1038/s41598-023-47579-7 (2023).
Sun, X. Y. et al. Transmutation of MAs and LLFPs with a lead-cooled fast reactor. Sci. Rep. 13 (1), 1693. https://doi.org/10.1038/s41598-023-29002-3 (2023).
Pilgrim, C. D. et al. Tuning aminopolycarboxylate chelators for efficient complexation of trivalent actinides. Sci. Rep. 13 (1), 17855. https://doi.org/10.1038/s41598-023-44106-6 (2023).
Download references
Author information
Authors and affiliations.
Institute of Resource Ecology, Helmholtz-Zentrum Dresden-Rossendorf, Bautzner Landstrasse 400, 01328, Dresden, Germany
Kristina Kvashnina
The Rossendorf Beamline at ESRF, CS 40220, 38043, Grenoble Cedex 9, France
BRGM, 3, Avenue Claude Guillemin, 45060cedex 2, Orléans, France
Francis Claret
ICSM, University of Montpellier, CEA, CNRS, ENSCM, Marcoule, France
Nicolas Clavier
Pacific Northwest National Laboratory, 902 Battelle Blvd, Richland, WA, 99354, USA
Tatiana G. Levitskaia
Massachusetts Institute of Technology, Cambridge, MA, USA
Haruko Wainwright
Idaho National Laboratory, Idaho Falls, ID, 83415, USA
Tiankai Yao
You can also search for this author in PubMed Google Scholar
Corresponding author
Correspondence to Kristina Kvashnina .
Ethics declarations
Competing interests.
The authors declare no competing interests.
Additional information
Publisher's note.
Springer Nature remains neutral with regard to jurisdictional claims in published maps and institutional affiliations.
Rights and permissions
Open Access This article is licensed under a Creative Commons Attribution 4.0 International License, which permits use, sharing, adaptation, distribution and reproduction in any medium or format, as long as you give appropriate credit to the original author(s) and the source, provide a link to the Creative Commons licence, and indicate if changes were made. The images or other third party material in this article are included in the article's Creative Commons licence, unless indicated otherwise in a credit line to the material. If material is not included in the article's Creative Commons licence and your intended use is not permitted by statutory regulation or exceeds the permitted use, you will need to obtain permission directly from the copyright holder. To view a copy of this licence, visit http://creativecommons.org/licenses/by/4.0/ .
Reprints and permissions
About this article
Cite this article.
Kvashnina, K., Claret, F., Clavier, N. et al. Long-term, sustainable solutions to radioactive waste management. Sci Rep 14 , 5907 (2024). https://doi.org/10.1038/s41598-024-55911-y
Download citation
Published : 11 March 2024
DOI : https://doi.org/10.1038/s41598-024-55911-y
Share this article
Anyone you share the following link with will be able to read this content:
Sorry, a shareable link is not currently available for this article.
Provided by the Springer Nature SharedIt content-sharing initiative
Quick links
- Explore articles by subject
- Guide to authors
- Editorial policies
Sign up for the Nature Briefing newsletter — what matters in science, free to your inbox daily.

- Nuclear Transparency Watch
Prevent and anticipate through transparency and participation
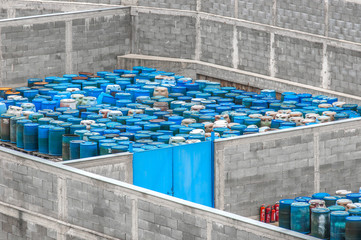
Three case studies on radioactive waste
9 December 2021
These are case studies in a larger report on radioactive waste and transparency, currently under preparation for the Euratom EURAD programme by Nuclear Transparency Watch. Publication was expected in October 2021.
Slovenia and croatia – radioactive waste, transparency, shared responsibilities, shared problems.
By Nadja Zeleznik – Nuclear Transparency Watch
Slovenia and Croatia share the nuclear power plant Krško (NEK) which was constructed as a joint venture during 1970-ties in the socialist Federal Republic of Yugoslavia as part of the larger nuclear programme on the use of nuclear energy. NEK is a two loop PWR Westinghouse (USA) design with all supporting infrastructure on site, including the buildings for radioactive waste and spent fuel management. Licensing was performed by the Republic Committee for Energy, Industry and Construction as the responsible authority in Slovenia. All other authorities were coordinated by this Committee, including the Expert committee on nuclear safety with its Technical Support Organizations. A safety report with safety analyses was mainly based on the provisions from USA NRC legal framework because the plant was of USA design. Trial operation was granted in 1981, in 1983 commercial operation started, and a license for normal operation was obtained in 1984 under Yugoslav and Slovene legislation.
A shared facility
Operator NEK d.o.o. is organized as a limited liability company in 100 % state ownership from entities of two republics: 50 % is owned by GEN energija d.o.o from Slovenia and 50 % by HEP d.d. from Croatia, both in 100 % ownerships by their states and the successors of the initial investors. The owners of NEK are equally responsible for ensuring all material and other conditions for safe and reliable operation of NPP, whereas the regulation and supervision of nuclear and radiation safety for NEK is the sole responsibility of the Republic of Slovenia. The regulatory framework for nuclear and radiation safety consists of the Ionizing Radiation Protection and Nuclear Safety Act with a set of regulations and decrees that are harmonised with international developments. All other legal requirements are incorporated in other legislation in Slovenia. The responsible authorities are primarily the Slovenian Nuclear Safety Authority (SNSA) within the ministry responsible for environment and the Slovenian Radiation Protection Administration (SRPA) within the ministry responsible for health.
After the breakup of Yugoslavia in 1991, NEK continued to operate under the legal framework of the Republic of Slovenia, although co-ownership with the Republic of Croatia was recognized and never argued but was not well defined under the new legal systems of both countries. Next to one major dispute on the energy supply which finished with lawsuits by both owners, the governments agreed to define in more details mutual relations regarding the status of NEK, its exploitation and decommissioning and adopted in 2001 the Agreement between the Government of the Slovenia and the Government of the Croatia on the Regulation of the Status and Other Legal Relations Regarding the Investment, Exploitation and Decommissioning of the Krško NPP (Intergovernmental Agreement – IA), ratified by both parliaments in 2003. The most vital points in the IA are the establishment of NEK decision making bodies, with as most important the Intergovernmental Commission (IC), in order to monitor the implementation of the IA, responsibilities in relation to the production of electricity, transmission, costs, recruitment, education, contractors and support for equal opportunities for workers. A very important part of the IA is devoted to decommissioning of NEK and radioactive waste and spent fuel management, where several provisions are agreed:
- Decommissioning of NEK, the disposal of all generated radioactive waste and spent fuel, as specified in the IAEA Joint Convention on safety of radioactive waste management and safety of SF management, is a joint obligation of the parties.
- Parties agree to provide an effective joint solution for the decommissioning and disposal of radioactive waste and spent fuel from an economic and environmental point of view.
- Programme of radioactive waste and spent fuel disposal: developed in accordance with international standards with the participation of NEK by responsible organizations (ARAO as waste management organisation in Slovenia and the Fund for financing the decommissioning of the Krško nuclear power plant in Croatia). The programme includes proposals for the possible division and takeover of radioactive waste and spent fuel, acceptance criteria for the disposal and assessment of the necessary financial resources, and deadlines for implementation and revised at least every five years.
- Decommissioning programme: includes the management of all radioactive and other wastes generated during the decommissioning, until their removal from the NEK site, an estimate of the necessary financial resources and deadlines for its implementation, revised at least every five years.
- The location of NEK may be used for temporary storage of radioactive waste and spent fuel for the rest of its lifetime.
- If parties do not agree on a common solution for the disposal of radioactive waste and spent fuel by the end of its regular lifetime (that is until 2023), they shall undertake removal of radioactive waste and spent fuel from the NEK site no later than two years thereafter (until 2025), each half. Further removal of radioactive waste and spent fuel will take place in accordance with the radioactive waste and spent fuel disposal programme and the decommissioning programme, at least every five years, unless otherwise specified by the approved programmes.
- Financing of the costs of the preparation of the decommissioning programme, the costs of its implementation, as well as the costs for the radioactive waste and spent fuel disposal programme shall be shared in equal parts.
R adioactive waste and transparency
For improvement of safety and due preparation of NEK lifetime extension, a dry spent fuel storage is under development with the construction license issued in December 2020. Under pressure from environmental organisations, an environmental impact assessment (EIA) was carried out, and NEK has to take into account also some measures and conditions to mitigate adverse effects including zero base monitoring before facility construction, protection of soil and water, and emergency preparedness. The Waste Manipulation Building intended for storage and further manipulation of radioactive waste in drums was constructed in 2018. This happened without EIA. NEK’s lifetime extension for 20 years, which is inevitably linked with radioactive waste and spent fuel generation, was initiated. SNSA took in 2012 a decision in principle, referring to the results of two Periodic Safety Reviews in 2023 and 2033. However, such an approach did not follow non-nuclear legislation and NEK had to file an application for lifetime extension to the responsible body ARSO in 2016. Only after an appeal from NGOs and a judgement from the Administrative Court in October 2020, ARSO decided that for the NEK lifetime extension an EIA is obligatory. The process will take several years, but information and participation will be assured.
The long-term radioactive waste and spent fuel management from NEK is defined in the Programme of NPP Krško Decommissioning and spent fuel and Low and Intermediate Level Waste (LILW) Disposal (DP) which was so far adopted with two revisions. The main purpose of the DP is to propose technical solutions, to estimate decommissioning and radioactive waste and spent fuel disposal costs for NEK, and to calculate annual instalments for devoted funds in Slovenia and Croatia. DP Rev.1 was approved by the Intergovernmental Commission, adopted by Slovenian government and Croatian parliament at the end of 2004. In 2011, the DP rev.2 was developed with new boundary conditions, including the option of NEK lifetime extension, but this was never adopted. There were no clear statements why there was no agreement. DP rev. 3 was developed again under new boundary conditions (like the NEK lifetime extension, dry SF storage as part of NEK’s operation, so only three projects were still to be addressed: NEK decommissioning, LILW disposal and SF disposal) and was adopted in 2020 by the same main authorities: the IC, the Slovenian Government and the Croatian Parliament. A joint LILW repository was rejected by the council of the municipality of Krško, so in the DP Rev. 3 two separate radioactive waste disposals are taken into account: one in Slovenia on the selected site Vrbina, next to NEK, and one in the potential radioactive waste centre Čerkezovac in Croatia, although the latter is still in the licensing process. Therefore, the division of operational and decommissioning radioactive waste is analysed and included in this revision, starting with the removal of existing waste from NEK in 2023. Regarding spent fuel disposal, a joint solution is still foreseen between the two states. During the development of the DP, no public participation took place and all decision making was entrusted to the Intergovernmental Commission and its advisory committees.
In between, the Slovenian LILW repository has evolved, and a site licence was obtained in 2010 for a modular silo version of the repository at the Vrbina, Krško site, which is just next to the NEK. Information and public participation were broader than required and during site selection local partnerships were functioning, although later ceased. In the EIA report, two silos were included, thus creating the possibility that the disposal contains all radioactive waste generated at NEK. The construction licencing procedure is now in its final stage, while in parallel an EIA procedure with prescribed public participation, including a public hearing and 30 days for comments and suggestions, and a transboundary EIA (including also Croatia) is performed.
Challenges of shared responsibility
Until the adoption of the IA in 2003, the management of different issues of NEK were also bringing disagreements between the co-owners. One of the major ones was the issue of costs for NEK operation and the related decommissioning, radioactive waste and spent fuel management, to be set in a dedicated fund. The dispute ended with lawsuits and with the decision that Slovenia had to pay a total of around 40 million € due to the non-supply of electricity to Croatia in 2002 and 2003.
After the adoption of the IA in 2003, relations have become much more defined with procedures on how to approach in case of divergences. For on-site radioactive waste and spent fuel management, a basic decision-making process is in place and no disagreement is reported publicly (e.g., in media). However, for several new radioactive waste and spent fuel buildings on site or even for the lifetime extension of the NPP, NEK tried to minimise public participation. EIA processes started only after successful appeals by NGOs, administrative court rulings and new decisions of ARSO: the EIA for the Waste Manipulation Building, an EIA process for the Dry Spent Fuel Storage, and an EIA for the NEK lifetime extension.
With regards to the long-term decisions for the radioactive waste and spent fuel management from NEK operation and future decommissioning, the issues are less conclusive and more complex. The main decision-making body defined in the IA is the Intergovernmental Commission. The basic documents that define the future decommissioning and disposal activities are the Programme of radioactive waste and spent fuel Disposal and Decommissioning, which should be developed every five years. The mechanisms for development of those programmes are also in place: two responsible organizations – ARAO and the Fund – with sufficient knowledge and resources for development of work, based on a Terms of Reference (ToR), adopted by the IC and further confirmed by the Slovenian Government and Croatian Parliament. However, the process of regular adoption of new revisions every five years was not successful. After the DP, Rev. 1, adopted in 2004, the Revision 2 of the DP scheduled to be adopted in 2009, although started on time, was never adopted. Only in 2020, Revision 3 of the DP was adopted, but the joint solution for LILW management was not agreed and two separate LILW repositories are planned for. The reasons for rejection of a joint LILW repository establishment were never set out in writing, but the basic principles as proposed by the advisory body to the IC (on safety of solutions, disposal of all radioactive waste in Slovenia and Croatia, optimization of costs and equal participation of entities from both countries) were already rejected at the level of the Krško municipality and were just taken over by the IC.
According to the IA, the decision making is limited to the official representatives of both countries, namely the members of the IC and its advisory body (this time called the Implementation Coordinating Committee), basically represented by appointed high ranking politicians or heads of responsible organizations. There is no other decision making foreseen, as programmes are seen as a kind of strategic documentation. However, there is a question whether such documents should also be also open for public participation (in terms of any kind of environmental assessment or other unofficial discussions) and would such broadening of transparency increase the acceptability of projects. Arguments for such public participation can be found in the Aarhus Convention, article 7, which obliges public participation for plans, programmes and policies related to the environment, the Kiev Protocol to the Espoo Convention on strategic environmental assessments and the EU Strategic Environmental Assessment Directive 2001/42/EC.
Typical for NEK activities, transparency in terms of nuclear safety and Waste Directive requirements remains an issue of concern: the approach used is to go for construction licenses to the Ministry of Environment and Spacial Planning, where SNSA provides consent for the nuclear safety and radiation protection part. Such an approach definitely shortens the procedure, but also excludes any public participation. Only lately we see a change, basically due to the appeals from NGOs to require EIA procedures for projects.
In relation to development of long term radioactive waste and spent fuel management solutions for NEK, the implementation of the IA is not so effective and successful, the functioning of the IC is limited. Members of the IC are changing with changing governments: the lead from each country is the responsible minister, a state secretary in the ministry and some other state officials. So the IC changes after each election. It would be important to stabilise future functioning of the IC and to think about professionalisation of the body. If the members would not change every two years (the current rate of government changes in Slovenia), they would be much more knowledgeable in the area, and also much more independent in decisions. Currently, the IC is perceived as a political body and also the broader context of relationships between the countries impacts its functioning (like the disputed border on the sea).
Transparency including information provision and public participation (not to mention access to justice) of developed programmes decided by the IC is really a weakness. Decisions are taken by the IC, on websites there is no further information on how decisions have been taken, the public is informed on press conferences about the outcomes. The programmes are published only after they are adopted and there is no public participation. However, individual projects (like the LILW repository) are going through all steps as prescribed in legislation, including an EIA process. The Law on environmental protection already now requires that for strategies or plans, a strategic environmental assessment (SEA) should be performed, also including public participation for important national strategies. Following the definitions in the Aarhus Convention, the Kiev Protocol to the Espoo Convention and the SEA Directive, the DP has to be understood as a national programme which directs radioactive waste and spent fuel management from NEK.
An open discussion on the shared option and a structured dialogue with interested parties from both countries would enable a more flexible approach in which disagreement could be addressed and potentially mitigated and solved.
Foreign radioactive waste treatment in Jaslovské Bohunice, Slovakia Transformation from a national to international treatment centre
By Michal Daniska (Nuclear Transparency Watch)
Since 2013 (at the latest) foreign LLW and VLLW has been treated at RW Treatment and Conditioning Technologies in Jaslovské Bohunice (RW TCT), Slovakia, mainly through incineration. Hundreds of tons of RW from the Czech republic, Italy and Germany have been incinerated or contracted for incineration at RW TCT (see the sec. 4.2.3 for details). The transformation of RW TCT from the exclusively national facility to an international RW treatment provider was done without prior consultation with, and approval by the public and municipalities which, according to, e.g., one of the mayors [1] might have found out about it only in 2018 (i.e., after approx. 5 years). The foreign RW share incineration varied between approx. 35-45% during 2015-2019 and exceeded 50% for the first time in 2020. Foreign RW treatment, especially by means of incineration, was originally categorically rejected by the vast majority of the affected municipalities. However, multiple municipalities later turned their position by 180 degrees on the condition, among others, that they received economic and non-economic incentives [2] . Unusually strong refusal arose also among the public, e.g., approx. 3 000 citizens signed a petition against capacity increase of RW TCT and demanding prohibition of foreign RW treatment in Slovakia. Meanwhile, the operator applied for an increase of the RW TCT treatment limits from 8343 to 12663 t/year in total (including an increase from 240 to 480 t/y by incineration) and a second incineration plant has been constructed. There is some evidence supporting an opinion that Slovakia itself does not need such an increase of treatment (or at least incineration) capacities and a suspicion that the second incineration plant might be purpose-built to better fit the specific RW from the Caorso NPP, Italy. The Slovak Atomic Act allows import, treatment and conditioning of foreign RW on condition that the radioactivity level of the imported RW equals the radioactivity level of the reexported (after treatment and conditioning) RW. Since the change of government in March 2020, the new Minister of Environment has been trying to ban foreign RW incineration by law. On 6 October 2021, the Slovak Parliament approved a bill which aims at banning future contracts for incineration of foreign RW on the Slovak territory. The already signed contracts for incineration of foreign RW from Italy (617 m 3 and 865 tons) and Germany (21.7 t) will not be affected by the bill [3] .
Historical context
RW TCT is a part of a larger nuclear site near Jaslovské Bohunice, Slovakia, that also includes NPP A1 and V1 (both being decommissioned), NPP V2 (in operation), interim SNF storage and other nuclear installations. In addition, a new nuclear reactor is planned in this locality (EIA process completed in 2016). NPP A1, commissioned in 1972, was the first NPP in the former Czechoslovakia. Being operated only for 5 years, NPP A1 was permanently shut down after two serious accidents in 1976 and 1977. Shortly after the process of decommissioning had slowly begun and continues currently. The core of the RW TCT was designed to ensure the process of treatment of RW produced during the decommissioning of NPP A1. As a result of gradual development, the RW TCT in its current state includes e.g., two incinerators for solid, liquid RW and saturated sorbents; facilities for super-compaction of solid RW; metallic RW remelting; fixed RW pre-conditioning; concentration of liquid RW; solid RW sorting; bituminisation and other installations. The first of the two incinerators is a shaft furnace type (as in Seibersdorf, Austria), was built between 1993-1999 and has been operated since 2000. The second incinerator has a rotary kiln, its project dates back to February 2017 at the latest, has been constructed between 2019-2021 and is going to be commissioned soon.
RW TCT is owned and operated by JAVYS ( Ja drová a vy raďovacia s poločnosť = Nuclear and decommissioning company), a state-owned stock company (the Ministry of Economy of the Slovak republic holds 100% of the company stocks). Originally, before JAVYS was founded in 2005, RW TCT belonged to Slovenské elektrárne (i.e., “Slovak power plants”) company. Prior to its privatisation in 2006 Slovenské elektrárne had been a state-owned company which operated all the power plants in Slovakia including the nuclear ones and the related infrastructure (e.g., RW and SNF management facilities). JAVYS was founded by separating it from Slovenské elektrárne in 2005. JAVYS was not a subject of privatisation and, as a result, has remained completely state-owned. At the time of its founding JAVYS consisted of selected nuclear assets in which the Italian ENEL company, the winner of the business competition for privatisation of the Slovenské elektrárne, was not interested. In addition to RW TCT, these assets included the NPP A1 and V1 (and their decommissioning), the Interim SNF storage (in Bohunice) and the National repository for LLW and VLLW in Mochovce. At the moment, JAVYS is also responsible for the deep geological repository project, holds the de facto monopoly position in interim storage of Slovak SNF, decommissioning and management of RW and, through a 51% share, takes part in the project of the new NPP in Jaslovské Bohunice.
Foreign radioactive waste and plans to increase RW TCT capacity
At RW TCT, the foreign RW is treated mainly by incineration which, until the second incinerator is commissioned, takes place exclusively at the first incinerator. The incineration of foreign RW dates back to 2013 when 8.8 tons of Czech RW were incinerated. In 2012 the volume of the incinerated Slovak RW reached its historical minimum after it had decreased from approximately 140t to 50t a year between 2007-2012. Gradually, the Nuclear regulatory authority of the Slovak republic (NRA SR) issued permissions for incineration of (1) 39.64t RW from NPP Temelín and Dukovany, Czech republic (31.10.2013); (2) 7t +16m 3 institutional RW from Italy (03.09.2015); (3) 145.2t RW from NPP Temelín and Dukovany, Czech republic (27.11.2015); (4) 800t ion-exchange resins in urea formaldehyde and 65t sludge from the decommissioned NPP Caorso, Italy (04.06.2018); (5) 21.7t institutional RW from Germany (22.01.2019) and (6) 617m 3 institutional RW from Italy (25.01.2019). Incineration of approx. 1600 tons of foreign RW (Czech rep., Italy, Germany) was contracted in total, out of which approx. 300 tons have already been incinerated between 2013 – 2020. In comparison, approx. 1100-1200 tons of Slovak RW were incinerated between 2007-2020. In the period 2015-2019 approx. 110-130t of RW in total were incinerated annually, out of which the Slovak RW represented 60-85t, the share of foreign RW at incineration oscillated between 34-46% (43-56 tons annually) and exceeded 50% for the first time in 2020. Although the current legal limit for RW incineration is 240 t/year, according to JAVYS it is in practice not technically feasible to incinerate more than 130-150 t/year of RW at the first incineration plant. In case the capacity increase (in case of incineration from 240 t/year to 480 t/year) is approved and the second incineration plant becomes operational, the volume of incinerated RW in practice may increase to approx. 420-460 t/year (i.e., approx. 3 to 5-fold increase if compared to the current state), and the foreign RW share at incineration might exceed 70% [4] .
The public did not participate in the authorization processes for import and incineration (treatment) of foreign RW in Slovakia held by NRA SR which resulted in the six permits mentioned above. The available information does not indicate that mayors of the affected municipalities were aware of the ongoing foreign RW incineration until about 2018 [5] . However, at least since 2014 the mayors have been considering the risk of such activities, although only as a theoretical option in the future. During various EIA processes, the municipalities regularly (as a precaution) expressed their disapproval of foreign RW treatment in the Jaslovské Bohunice locality until 2019. Nevertheless, we have not found any evidence that the municipalities were explicitly notified of the ongoing foreign RW treatment (incineration) during the EIA processes before 2017/2018. The EIA process “ RW processing and treatment technology by JAVYS, a.s. at Jaslovské Bohunice location ” (December 2012 – November 2014), during which the already existing and operated RW TCT were assessed for the first time on the basis of modern EIA legislation, might be used as a particular example. During a public hearing, which took place in March 2014, the mayors directly and indirectly asked about the possibility of treatment of RW from locations other than Jaslovské Bohunice. In response JAVYS did not inform about foreign RW treatment (e.g., incineration) that had already been carried out (8.8 tons RW from the Czech Republic incinerated in 2013) or that which had already been contracted. The statement of JAVYS was formulated in a conditional way, as if the treatment of RW for other companies (aside from RW from Mochovce NPP) was not a reality yet. Although it was admitted that contracts for RW treatment and conditioning were being sought, it was not directly mentioned that the RW would come from abroad. In addition, “ foreign RW treatment ” was not even once explicitly mentioned, neither in the EIA plan, EIA report nor in the minutes from the public hearing. Finally, the Environmental Impact Statement (EIS) from this EIA process (issued in November 2014, with minor changes valid until today) explicitly states that RW TCT serves the treatment and conditioning of VLLW, LLW and ILW from (1) decommissioning of the Slovak NPPs A1 and V1; (2) operation of Slovak nuclear installations; or (3) institutional RW (IRW) and captured RW (CRW). The list of purposes does not explicitly mention treatment (incineration) of foreign RW. In March 2021 the Slovak Ministry of Environment stated that “ the ongoing foreign RW treatment (incineration) is inconsistent ” with the EIS mentioned above [6] . However, the treatment of foreign RW at RW TCT continues.
It was not until the beginning of 2018 that information on the treatment (incineration) of foreign RW resonated for the first time among municipalities and a part of the public. There were two main sources – (1) the press conference of then opposition MPs Mr. Igor Matovič and Mr. Marek Krajčí about incineration of RW from the decommissioned Italian NPP Caorso and their failed attempt to ban incineration of foreign RW by law (February 2018) followed by (2) publishing the EIA plan “ Optimisation of treatment capacities of radioactive waste treatment and conditioning technologies JAVYS, a.s. at Jaslovské Bohunice ” (March 2018) where foreign RW treatment was mentioned among the purposes of RW TCT. However, the treatment and especially incineration of foreign RW gained more significance and repeated media attention only in the middle of 2020, after the February 2020 elections and the consequent change of government (Mr. Matovič and Mr. Krajčí became the Prime Minister and the Minister of Health, respectively).
EIA processes
The environmental impact assessment (EIA) project “ Optimisation of treatment capacities of radioactive waste treatment and conditioning technologies JAVYS, a.s. at Jaslovské Bohunice ” stands for capacity increase of RW TCT, from 8343 to 12663 t/year in total (all technologies). It also covers the second incinerator and an increase of the incineration limit from 240 to 480 tons per year (corresponding to the real incinerated volume increase from approx. 130t/y, the technical limit of the first incinerator, to approx. 420-460t/y if both incinerators are in operation); capacity increase of the metallic RW remelting from 1000 to 4500t/y, and so on. In April 2018, the vast majority of the municipalities categorically refused foreign RW treatment, especially incineration, and the proposed RW TCT capacity increase, reasoned with arguments of (protection of) a healthy environment for their citizens (not by unmet financial requirements). An individual EIA process for the second incinerator was only launched in September 2018 under the name “ Optimisation of incineration capacities of the nuclear installation RW Treatment and Conditioning Technologies ”, thus accelerating the authorization process of the second incinerator. JAVYS justified the second incinerator with an expected approx. 50% increase in production of domestic combustible RW [7] in 2020-2023 and the necessity to have an operational incinerator capacity within the assessed limit of 240 tons/year (the technical limit of the first incinerator is approximately 130t/y only) in order to “ meet emerging requirements for RW treatment from decommissioning and also from the operation of NPPs in the Slovak Republic ”. Based on this justification, the majority of the municipalities approved the second incinerator providing that the limit 240t/y (for both incinerators together) is preserved and no foreign RW is incinerated at the second incinerator. These conditions, explicitly accepted by JAVYS, were transposed into the final ruling issued in this individual EIA process. Under these conditions, the municipalities did not obstruct the authorisation process and already in June 2019 the NRA SR could have issued a construction permit and the construction of the second incinerator could have begun.
On 24.03.2021 the Ministry of Environment of the Slovak republic issued the EIS no. 417/2021-1.7/zg from the EIA process “ Optimisation of treatment capacities of radioactive waste treatment and conditioning technologies JAVYS, a.s. at Jaslovské Bohunice ” which, however, has not entered into force yet due to appeals lodged. This EIS approved the proposed capacity increase and set no restrictions on foreign RW treatment. The Ministry of Environment argued that 1.) it cannot interfere with or restrict business activities if significantly negative impact on the environment had not been demonstrated and 2.) there is a constitutional right to engage in business and other gainful activity [8] . Once the EIS from the EIA process “ Optimisation of treatment capacities of radioactive waste treatment and conditioning technologies JAVYS, a.s. at Jaslovské Bohunice ” comes into effect, the EIS from EIA process “ RW processing and treatment technology by JAVYS, a.s. at Jaslovské Bohunice location ” and the ruling from the individual EIA process for the second incinerator expire. Therefore, the restriction prohibiting incineration of foreign RW at the second incinerator will expire as well, unless the EIS no. 417/2021-1.7/zg is amended as a result of the appellate procedure and such restriction is added to the EIS.
It is important to point out that JAVYS agreed to exclude foreign RW treatment at the second incinerator in December 2018, i.e., in a situation when the EIA process “ Optimisation of treatment capacities of radioactive waste treatment and conditioning technologies JAVYS, a.s. at Jaslovské Bohunice ” was already in progress and permits for incineration of foreign RW valid today had already been issued by NRA SR or the permits had already been requested. Also, the condition prohibiting incineration of foreign RW will be de facto applied only after the second incinerator is commissioned.
In 2019-2020 the volumes of incinerated Slovak RW reached approx. 60 tons per year (1/8 of the proposed increased limit 480t/y) which means an approx. 25% decrease if compared to the period 2016-2018 (approx. 80-85 t/y). This data does not seem to be in accord with the statement of JAVYS from 17.12.2018 when it expected an approx. 50% increase in Slovak RW production from NPP decommissioning in the period 2020-2023 and used it as the primary reason for justification of the second incinerator [9] . Also, according to the National policy for management of SNF and RW in the Slovak republic (2015), the current capacity of RW treatment lines (i.e., without the second incineration plant) is sufficient (with reserves) for treatment of RW from both operation and decommissioning of the Slovak nuclear installations. These conclusions are consistent with the data volumes of incinerated Slovak RW (60-85 t/y in 2015-2019) which is significantly below the technical capacity of the first incinerator (approx. 130t/y).
There were two public hearings during the EIA process “ Optimisation of treatment capacities of radioactive waste treatment and conditioning technologies JAVYS, a.s. at Jaslovské Bohunice ” – on 26.08.2019 and 16.12.2019. Among others, JAVYS declared on 26.08.2019 foreign RW treatment as “ a complementary activity ” (compare this to a 34-46% share of foreign RW at incineration in 2015-2019 and a possible expected increase to over 70% in the future); on 16.12.2019, JAVYS, among others, claimed that foreign RW share at incineration was 12% only The public obtained data about volumes of incinerated domestic and foreign RW only in the middle of 2020 (e.g., through a request of information sent to NRA SR [10] ).There have been attempts to obtain more detailed data about incineration of foreign RW (e.g., activity streams, production and management of the secondary RW) which, however, have led to very limited success.
Currently, after a position change in 2019, the majority of the affected municipalities and the association of municipalities explicitly approved both the RW TCT capacity increase and foreign RW treatment (up to 30% share) on the condition (among others) that new economic and non-economic incentives for municipalities in the region are established. Two county towns (Hlohovec and Piešťany) and some other municipalities continue opposing the project. Approx. 3000 citizens signed a petition against capacity increase of the RW TCT and demanding a prohibition of foreign RW treatment in Slovakia.
The affected villages that now consent to the project received 10000€ each from JAVYS in December 2019. On the contrary, the opposing affected villages received only 2500€ or 0€ [11] . The following year in December 2020, after this fact was published in the media, all 9 affected municipalities received 10000€ each from JAVYS.
The Caorso contract
The Caorso contract for incineration of more than 30-year-old 800 tons of ion-exchange spent resins in urea formaldehyde and 65 tons of radioactive sludges (5881 tanks) from the shutdown Italian NPP Caorso, holds an exceptional position among the 6 contracts for foreign RW incineration at RW TCT. The main reason is the allegedly challenging nature of this RW is that it is said to lead to difficulties during incineration in the shaft furnace of the first incinerator and a suspicion that the second incinerator with a rotary kiln might be purpose-built to better fit the RW from Caorso and thus overcome these difficulties [12] . These doubts can be supported by (1) the original construction contract stating that the second incinerator must (with special regards) be capable of incineration of ion-exchange spent resins in urea-formaldehyde which shall be proven by successful hot tests with 100 tons of ion-exchange resins in urea-formaldehyde and 20 tons of other RW. In May 2021 the NRA SR explicitly confirmed that “ exactly the urea-formaldehyde resin represents the foreign RW ” [13] ; (2) the Caorso contract was signed in 2015, but hot tests at the first incinerator took place only in 2019, after a brand new pre-conditioning line was commissioned; (3) the hot tests at the first incinerator took almost half a year (21.01.2019 – 02.07.2019) and large volumes (43 tons reduced by preconditioning to 15,5 tons) were incinerated; (4) almost a threefold reduction of RW mass through pre-conditioning; (5) the contracting process for construction of the second incinerator took place 20 months after the Caorso contract was signed (February 2017 vs. June 2015); (6) incineration at the second incinerator does not result in alpha cross-contamination of the (foreign RW) ashes; (7) the residual capacity of the first incinerator (i.e. after incineration of the Slovak RW) is approx. 60-80 t/y, which does not seem to be sufficient to meet the Caorso contract deadline in 2023 (assuming the contract volume 865 ton and also other contracts, e. g. 617m 3 of institutional RW from Italy).
However, a direct connection between the second incinerator and the Caorso contract has not been confirmed, neither by JAVYS nor by the nuclear regulator NRA SR. Transparency is deficient not only in the clarification of the relation between the Caorso contract and the second incinerator (and the preconditioning line), but also in the Caorso contract itself. The Caorso contract was, after a significant portion of relevant data had been redacted, published online in November 2020.
Prohibition of future contracts for foreign RW incineration by law
Since the change of government in 2020, the new Minister of Environment, Mr. Ján Budaj, has been trying to ban foreign RW incineration by law. These efforts encountered a significant obstacle represented by huge financial penalties in case the Caorso contract is terminated. Different positions of the Ministries of Environment and Economy on this topic led in February 2021 to a compromise proposal that the ban would not affect the already signed contracts for foreign RW incineration. A corresponding legislative bill was submitted in the Slovak parliament at the end of May 2021. On 6 October 2021, after significant changes were made to the wording of the bill at an advanced stage of discussion in the Parliament, the Slovak Parliament approved a bill which aims at banning future contracts for incineration of foreign radioactive waste on the Slovak territory [14] . After the president of the Slovak republic signed the bill on 25 October 2021, which will come into effect on 1 January 2022. The already signed contracts for foreign RW incineration will not be affected by the bill. This concerns 617 m3 and 865 tons of RW from Italy and 21.7 t from Germany, i.e. amounts that significantly exceed the volumes of incinerated domestic RW (about 60 – 85 tons annually during the period 2016-2020).
Challenges related to the foreign waste
Correct and complete impact assessment of the foreign RW treatment is a challenging task. For example, tracking down where all foreign radionuclides might end up could be highly relevant. One of the reasons is that the ratio of radioactivity retained in ash after incineration compared to radioactivity of the input RW is variable and significantly below 100% (on average approx. 60% in the period March 2020 – October 2021). At the same time wastewater from wet filtration of flue gases from RW incineration, which might contain a significant share of foreign radionuclides, ends up permanently in the RW repository in Mochovce. In order to analyse the fraction of foreign radionuclides that remain in Slovakia and how these missing radionuclides are replaced by Slovak radionuclides the public requested, mostly unsuccessfully, data about radioactivity streams during RW preconditioning, incineration and post-treatment (e.g., how much radioactivity is carried to the wastewater) and the production of secondary RW. This data is crucial in order to analyse the impact of the foreign RW treatment, especially by incineration. However, when requested, the nuclear regulator NRA SR could not provide (did not have) detailed data about activity streams in the treatment process. The data cannot be obtained from JAVYS either, since it claims not to be a liable entity according to the Slovak Freedom of Information Act.
Financial impacts should be assessed in detail as well. For example, the foreign RW owners do not participate in the future decommissioning of the RW TCT (especially the incinerators and the pre-conditioning line). The corresponding costs are expected to be covered by the National Nuclear Fund that collects money from Slovak electricity consumers. It could be worth analysing whether the Slovak taxpayers do not subsidise the foreign RW treatment in any (hidden) way (incl. construction, operation and future decommissioning costs, indirect costs – e.g., if the incinerator lifetime was negatively affected by the foreign RW treatment).
One can also argue that foreign RW treatment might challenge the ALARA principle. Slovakia is not legally or morally responsible for foreign RW, so it is reasonable not to incinerate/treat it and thus avoid any kind of unnecessary negative effects or risks. The Public Health Authority of the Slovak republic, Section of radiation protection justified its 2017 legislative proposal to ban foreign RW incineration by this argument.
Findi ngs and conclusions
The crucial issues here are transparency, public access to information, evidence-based decision making and effective public participation, which, among others, represent some of the key principles of the Aarhus Convention and the European Council Directive 2011/70/EURATOM. We consider it important to take into account that JAVYS is not a private but state-owned company and that most technologies of the RW TCT received necessary permits when the public and the municipalities implicitly assumed that RW TCT served management of the Slovak RW only and RW from decommissioning of NPP A1 in particular. First of all, the public discussion about foreign RW treatment should have taken place prior to RW treatment services being possibly offered to foreign customers, not years after foreign RW treatment in Slovakia started. The eventual ongoing discussion, which was initiated mainly by the public and the municipalities, is strongly affected by the risk of huge financial penalties in case the already signed contracts are terminated. This significantly reduces the set of options (de facto) available for discussion and subsequently impacts the results.
The second important issue are the difficulties in access to (objective and complete) information, information verification and consulting with independent experts in the case of the public and the municipalities. In practice the main source of information about activities at the nuclear site J. Bohunice for the general public are the corresponding EIA processes, since the EIA documentation is easier-to-read for non-experts, is published online and often also the public hearings take place in the affected municipalities. On the other hand, documentation from processes held by the nuclear regulator NRA SR is expert-oriented, can be accessed usually only via physical inspection and sometimes is even declared confidential. However, even in the EIA processes, the effectiveness of public participation is limited by information asymmetry between the public and municipalities on one hand, and the project proposer on the other. In case of nuclear installations, this asymmetry is further enhanced because of higher complexity of the problem. Due to limited time, expertise and financial resources the public and municipalities are reliant mostly on information provided by the project proposer, either in the EIA documentation or in reactions to additional questions (raised e.g., during the public hearing). Consultations with independent experts appear to be a theoretical option only, not only because of short procedural deadlines and financial constraints, but also due to a lack of suitable independent nuclear experts and/or insufficient free capacities of these experts. Even the Ministry of Environment failed while attempting to obtain an additional independent (expert) opinion on the EIA report within the EIA process “ Optimisation of treatment capacities of radioactive waste treatment and conditioning technologies JAVYS, a.s. at Jaslovské Bohunice ” in autumn 2020 [15] .
Effective public participation in the decision-making process requires that the public and municipalities are provided with correct and complete information about the project, its impacts and purpose as well as tools for easy information verification. The public should not be dependent on extensive and time-consuming investigation and information verification based on independent sources. The situation is negatively affected by the fact that JAVYS claims not to be a liable entity with respect to the Slovak Freedom of Information Act. This is difficult to understand, since this company is state-owned, carries out a public service and receives millions of euros from the public budget (through the National Nuclear Fund) each year, de facto holds a monopoly position in management of RW and SNF in Slovakia and, on top of that, it is also responsible for the project of the Slovak deep geological repository.
If the decision-making process is to be evidence-based, the project proposer shall be required to support all claims by objective and verifiable data.
Besides the deficiency in transparency and public participation and limited public access to information, the challenges related to the foreign RW treatment include (1) missing publicly available analyses of radioactivity streams, secondary RW production and corresponding data on the fraction of foreign radionuclides that remain in Slovakia and how these missing radionuclides are replaced by Slovak radionuclides; (2) non-participation of the foreign parties in the future decommissioning of RW TCT and in the legal responsibility in case of accidents or other indirect impacts; (3) missing publicly available detailed financial analyses including also of all indirect costs (it could be worth analysing whether the Slovak taxpayers do not subsidize foreign RW treatment in any (hidden) way); (4) reasonable doubts about the need of the second incinerator (in perspective of the Slovak needs) and clarification of the relation between the Caorso contract and the second incinerator (and the preconditioning line); (5) possible conflict of interests – e.g. some members of municipal councils employed at JAVYS; (6) financial power asymmetry between the proposer and the public. The distribution of substantial financial benefits from JAVYS to the affected municipalities in 2019 is highly correlated to the (dis)approval of the proposed RW TCT capacity increase by these municipalities; (7) law enforcement – the Ministry of Environment confirmed that “ the ongoing foreign RW treatment (incineration) is inconsistent ” with the still valid EIS. However, the treatment of foreign RW at RW TCT continues.
[1] “ We found out about it [foreign RW treatment] in either 2018 or 2019, I am not sure. ” A statement made by Mr. Gilbert Liška, the mayor of the municipality Veľké Kostoľany in the investigative videoreportage broadcasted on 15.06.2020 (part of “Reportéri RTVS” series) available at: https://www.rtvs.sk/novinky/zaujimavosti/227377/budeme-na-slovensku-spalovat-este-viac-odpadu (time 03:55-04:03)
[2] See, e.g., a statement of selected municipalities (in Slovak) available at https://www.jaslovske-bohunice.sk/evt_file.php?file=26988&original=stanovisko_obci.pdf and resolutions no. 2/28.10.2019, 3/28.10.2019 and 4/28.10.2019 of the Council of the Association of the municipalities in the region of the Bohunice NPP
[3] https://www.nuclear-transparency-watch.eu/activities/radioactive-waste-management/slovak-parliament-approved-a-bill-to-ban-future-contracts-for-incineration-of-foreign-radioactive-waste-according-to-the-slovak-ntw-members-some-concerns-still-remain.html
[ 4] During the period January 2021 – October 2021 94.78 and 37.81 tons of foreign and Slovak RW, respectively, were incinerated. i.e. the foreign RW share at incineration was 71.49% in this period.
[ 5] See, e.g., the statement of Mr. Gilbert Liška, mayor of the municipality Veľké Kostoľany, “ We found out about it [foreign RW treatment] in either 2018 or 2019, I am not sure ” made in the investigative videoreportage broadcasted on 15.06.2020 (part of “Reportéri RTVS” series) available at https://www.rtvs.sk/novinky/zaujimavosti/227377/budeme-na-slovensku-spalovat-este-viac-odpadu (time 03:55-04:03) and a statement of mayors of the affected municipalities dated 15 October 2018 demanding list of permits for incineration of foreign RW at RW TCT issued by the NRA SR and categorically refusing the foreign RW incineration (see p. 5-6 of the ruling no. 2764/2019-1.7/zg-R available (in Slovak) at: https://www.enviroportal.sk/eia/dokument/287520).
[6] See p. 90 of the English version of the EIS available at https://www.enviroportal.sk/eia/dokument/326075 or p. 86 of the Slovak version available at https://www.enviroportal.sk/eia/dokument/323308
[7] more precisely (according to a statement of JAVYS dated 17.12.2018): 50% increase in production of RW from NPP decommissioning combined with the start of production of combustible RW from operation of (to be commissioned) NPP Mochovce blocks 3 and 4 from 2020 onwards, i.e. 50% increase in the number of operated reactor blocks in Slovakia – from 4 to 6. However, the reactor blocks Mochovce 3 and 4 have not been commissioned yet.
[8] See p. 76 of the English version of the EIS available at https://www.enviroportal.sk/eia/dokument/326075
[9] Since the reactor blocks Mochovce 3 and 4 have not been commissioned yet, the missing contribution to increase in production of RW from NPP operation due to these blocks, originally expected by JAVYS in 2018, is not addressed here.
[10] The NRA SR responded to the request of information by the letter no. 3921/2020 dated 19.05.2020
[11] See https://www.rtvs.sk/novinky/zaujimavosti/227377/budeme-na-slovensku-spalovat-este-viac-odpadu at 11:22-12:50
[12] E.g., (1) news article dated 12.08.2020 available at https://www.aktuality.sk/clanok/813522/stali-sa-zo-slovakov-pokusne-mysi-olano-prudko-otocilo/ (translated from Slovak): “ Sceptics are convinced that JAVYS needs the new incineration plant, because the sludges from Italy cannot be incinerated at the old one . Even people who have been employed in the nuclear energy sector for years have no doubts about it. ”; (2) statement of the mayor of Veľké Kostoľany (one of the 9 affected municipalities) in the investigative videoreportage broadcasted on 15.06.2020 (part of “ Reportéri RTVS ” series) available at https://www.rtvs.sk/novinky/zaujimavosti/227377/budeme-na-slovensku-spalovat-este-viac-odpadu (time 04:27-04:46): “ We suppose that the new incineration plant is being purpose-built, since the RW imported from Italy cannot be incinerated at the old one ”; (3) official statement of the town Piešťany dated 28.04.2020: “ At the time of signing the contract, it became apparent that treatment of this type of RW at the old incineration plant would be very difficult. ”
[13] see NRA SR ruling no. 164/2021 P dated 24 May 2021, p. 20
[14] https://www.nuclear-transparency-watch.eu/activities/radioactive-waste-management/slovak-parliament-approved-a-bill-to-ban-future-contracts-for-incineration-of-foreign-radioactive-waste-according-to-the-slovak-ntw-members-some-concerns-still-remain.html
[15] See p. 49 of the English version of the EIS available at https://www.enviroportal.sk/eia/dokument/326075
DU Exports to Russia – A case of lack of transparency and research [1]
By Jan Haverkamp (Greenpeace, WISE)
From 1996, the uranium enrichment facilities URENCO Almelo (Netherlands) and URENCO Gronau (Germany) regularly sent shipments of depleted uranium (DU) in the form of UF 6 (uranium hexafluoride) to TENEX, later TVEL, in Russia, where this was stored in the open air in Seversk in the Krasnoyarsk region. Protests in Europe then halted these transports in 2009. TVEL is since 2007 a subsidiary of the Russian nuclear giant Rosatom. URENCO carries out enrichment for nuclear fuel production from natural uranium to low-enriched uranium for clients all over the world and has facilities in the Netherlands, Germany and the UK.
In 2019 and 2020, these transports were resumed from the enrichment facility of URENCO Gronau and URENCO UK in Capenhurst.
URENCO Almelo currently has a permit for export, but does not use it. Its DU is sent to France for conversion into stable U 3 O 8 (depleted tri-uranium-octo-oxide or uranium oxide), which is returned to the Netherlands and handed over to the waste management organisation COVRA for interim storage in the VOG facility, awaiting final disposal after 2100.
The claim is that the DU is sent to TENEX, later TVEL, for re-enrichment to natural level and reuse of the resulting double depleted uranium (DDU). Rosatom furthermore claims [2] that DDU and DU are used industrially and that the UF 6 also delivers fluorine for reuse purposes. It furthermore, describes in detail how it wants to convert its UF 6 stockpile into uranium oxide for waste treatment before 2057.
Our conclusion is that this form of TENORM (technically enhanced naturally occurring radioactive material) should be considered in principle as a waste material, for which full transparency should be assured over its complete chain of management, also when a limited amount of the material may be used as resource. Research on optimization of the management pathways should be part of European research programmes like EURAD.
Our central observations are:
- The involved DU is in Russia not a resource in the sense of sustainable recycling – that is, it is not 100%, nor for a majority of it, recycled and reused.
- The ownership structure of this export of DU to Russia hides this fact. Rosatom / TVEL has taken ownership of the material after export and with that, the material is out of sight of URENCO, its hosting EU Member State (MS) Germany, and of Euratom. It is today impossible for Euratom, Germany or URENCO to confirm whether the material is indeed reused in any form or not.
- Resulting radioactive waste from any management operation should be returned to the country of origin (as happens in the case of reprocessing of spent nuclear fuel). This does not happen in this case.
- Material that is not reused or recycled within a reasonable timeframe constitutes waste and has to be treated as such from the start. It falls under radioactive waste as defined in 2011/70/Euratom and the treatment of DU – whether within or outside the Union – should be considered as waste management.
- This implies that there should be a clear description of incurred streams of all treatment pathways in line with 2011/70/Euratom – and decisions on these treatment pathways should all be based on 2011/70/Euratom and complete knowledge of these pathways, irrespective of whether this treatment takes place within the Union or outside.
L aw and transparency
There is no transparency about the pathways of management of the DU exported by URENCO to Russia. There are claims of reuse on the Russian side, [3] but there are no clear descriptions of streams and involved amounts.
The Euratom radioactive waste directive defines radioactive waste as: “radioactive material in gaseous, liquid or solid form for which no further use is foreseen or considered by the Member State or by a legal or natural person whose decision is accepted by the Member State, and which is regulated as radioactive waste by a competent regulatory authority under the legislative and regulatory framework of the Member State;” (art. 3(7) 2011/70/EURATOM).
Article 4(2) of the directive states: “Where radioactive waste or spent fuel is shipped for processing or reprocessing to a Member State or a third country, the ultimate responsibility for the safe and responsible disposal of those materials, including any waste as a by-product, shall remain with the Member State or third country from which the radioactive material was shipped”.
Article 4(4) of the directive explains in more detail: “ Radioactive waste shall be disposed of in the Member State in which it was generated, unless at the time of shipment an agreement, taking into account the criteria established by the Commission in accordance with Article 16(2) of Directive 2006/117/Euratom, has entered into force between the Member State concerned and another Member State or a third country to use a disposal facility in one of them.
Prior to a shipment to a third country, the exporting Member State shall inform the Commission of the content of any such agreement and take reasonable measures to be assured that:
(a) the country of destination has concluded an agreement with the Community covering spent fuel and radioactive waste management or is a party to the Joint Convention on the Safety of Spent Fuel Management and on the Safety of Radioactive Waste Management (‘the Joint Convention’);
(b) the country of destination has radioactive waste management and disposal programmes with objectives representing a high level of safety equivalent to those established by this Directive; and
(c) the disposal facility in the country of destination is authorised for the radioactive waste to be shipped, is operating prior to the shipment, and is managed in accordance with the requirements set down in the radioactive waste management and disposal programme of that country of destination.”
Furthermore, the Euratom directive obliges in art. 10 transparency concerning radioactive waste.
In Russian law, the definition of radioactive waste is “ materials and substances not subject to further use, and equipment, articles (including spent ionizing radiation sources), in which the content of radionuclides exceeds the levels established in accordance with the criteria defined by the Government of the Russian Federation ” (clause 8, article 3). [4] This is a much more shady definition, whereby the issue of responsibilities for waste material and waste as by-product are not defined. It may therefore well be that where there is responsibility for exported DU for the state of origin under 2011/70/Euratom, there is none under Russian law.
Transfer of ownership and responsibility
In the current set-up, ownership of this TENORM is transferred from URENCO to TVEL / Rosatom. Nevertheless, 2011/70/EURATOM stipulates that “ the ultimate responsibility for the safe and responsible disposal of those materials, including any waste as a by-product, shall remain with the Member State or third country from which the radioactive material was shipped”. Logically, because URENCO Gronau does not reuse this material, for URENCO Gronau and Germany, this depleted uranium in the form of UF 6 is a waste material for which it has ultimate responsibility – also when others claim (but not prove in the form of accountable and accounted for pathways) use as resource. After all, ‘ultimate responsibility’ when the material is (partially) not reused in any way but ends de facto as waste, can never be shed. This has several consequences:
- The transfer of ownership does not release the Member State from which the material was shipped (e.g., Germany) from its ultimate responsibility for the depleted uranium and waste as by-product when it is not actually reused;
- For that reason, the Member State needs to have a full, proven and accountable overview of whether the material is indeed reused or not, and what is happening with all waste fractions, including by-products;
- The Member State should provide full (public) transparency about this – including the actual chains of handling – and the Member State should be involved in reducing the risks of that handling;
- The Member State should be involved in research about the handling of this stream of TENORM, in spite of (debatable!) claims that want it to be labelled as resource.
Resource or waste
Russia currently holds more than 1 million tonnes of DU in the form of UF 6 , around half of the world’s stockpile (Nikitin, 2020). It is unclear how much DU is held in stabilised form (in Russia, mainly DU 3 O 8 – depleted tri-uranium-octo-oxide). Rosatom is planning to convert its complete stockpile of UF 6 to DU 3 O 8 before 2057. Re-enrichment after that date would require re-conversion into UF 6 .
To determine whether the to Russia exported DU may be considered to be a resource (in that case falling out of the scope of the Euratom radioactive waste directive), it is important to establish how much of the material is indeed reused for other purposes, which waste by-products are produced and which part remains for how long in storage without being reused.
Besides the earlier mentioned publication from Bellona / Rosatom, there is no public information available about this, nor any publicly available formal plans from TVEL / Rosatom.
According to Nikitin (2020), under Russian law, the material is seen as a “strategic reserve for the existing nuclear power industry” because of the possibility of re-enriching the material or use in fast breeder reactors.
From different sources, the following potential uses of DU can be found:
- Reuse of fluorine
Nikitin (2020) puts a large emphasis on this. This is only possible when the uranium in the UF 6 is stabilised in the form of uranium-oxides and the fluorine is extracted. The resulting (radioactive) uranium would then only be further useful in metallic form (weapons, balancing, other) and breeder reactors, but not for re-enrichment. Given the fact there is no market lack of fluorine, it is highly unlikely that UF 6 for that reason would be kept as “strategic reserve” and hence justify labelling the DU as resource. Basically: declaring UF 6 a resource because of the need for toothpaste is nonsense.
- Re-enrichment to natural level
Given the limited availability of natural uranium in commercially viable extractable ores, re-enrichment of DU could squeeze out some more uranium for use in the nuclear fuel chain. Officially, re-enrichment is listed as justification for the export of DU by URENCO, and URENCO receives natural uranium back in return for the delivered DU. [5] It is unclear, however, whether this is indeed re-enriched DU or whether this is an equivalent of non-enriched natural uranium. Given the extremely energy intensive nature of enrichment, it is for cost reasons likely to be the latter. When typical 0,2 – 0,25% DU from the enrichment facility of URENCO is re-enriched by TVEL to a level of 0,72%, 1 ton of depleted uranium yields around 0,25 tons of enriched uranium (natural level) and 0,75 tons of double-depleted uranium. [6] This means that ¾ of the exported DU will remain in Russia in the form of double-depleted UF 6 , whilst maximally ¼ can be reused again by URENCO as natural level uranium for further enrichment purposes. URENCO then, in turn, delivers from these 0,03 tons of enriched uranium (nuclear fuel level) and another 0,22 tons of DU, which could yield another 0,06 ton of natural uranium and 0.007 ton of enriched uranium, etc. After many cycles, re-enrichment of DU could yield in total around 4% of the initial DU as fuel for nuclear power. 96% remains behind as double depleted uranium.
- Use of DU in breeder reactors
Rosatom has declared on several occasions that the DU is to be used as plutonium breeding resource for its breeder reactors. It is currently operating two fast breeder reactors in Beloyarsk. In its Belona paper, it states that the DU is to function as a reserve for the next millennia for its ‘” fast” energy industry [7] . However, Rosatom already has an enormous stockpile of DU from its own sources, sufficient for covering many centuries of use in the theoretical case it would decide to continue to expand this extremely expensive way of electricity generation. For all substances that currently would fall under the definition of waste, one can dream up some kind of reuse in millennia from now, but they remain waste. Normally spoken, when a substance cannot be used within one generation – be it either because of lack of technology to reuse it or because of abundance – it is usually considered waste. Substances like paper, aluminium or steel have a reuse cycle within several years after being discarded. For that reason, fictional reuse in millennia from now cannot be used as an argument. As soon as next generations will have to take care of the management of material with toxic, radiotoxic or otherwise problematic properties, this material should be considered waste that is handed over to those next generations. Next to that, given the fact that fast breeder technology has proven so far to be an extremely expensive and risky way to generate electricity (accident risk, proliferation risk), there is a very realistic chance that nothing or only a minute part of Rosatom’s current stockpile of DU would indeed ever be used in fast breeder reactors.
- use of DU for DU weapons
Rosatom (in Nikitin (2020)) does not mention this potential use of DU. It is unlikely, however, that Russia is not using DU in production of armour-piercing weaponry, similar to that used by NATO and the US during the wars in Bosnia and Iraq. It may be that Rosatom does not mention this, because potential dual use of material would make it fall under EU export restrictions to Russia. [8] Apart from this dual-use problem, it is unlikely that reuse in the weapon industry would amount to more than a few percent of the total stockpile of DU currently available in Russia. DU from the EU would for that reason continue to expand the stockpile and should not be considered to be reused this way.
- use of DU for balancing purposes (ships, aircraft)
Also, this potential reuse of DU is not mentioned by Rosatom (in Nikitin (2020)). The reasons are probably comparable with the use for DU weapons: potential military dual-use and the very minute amounts necessary in comparison with the available stockpile.
- use in radiation resistant concrete
Rosatom (in Nikitin (2020)) does mention ‘the manufacture of special radiation-resistant concrete’. From the description it remains unclear how much DU is used for this purpose in Russia, but the description of ‘casks and protective screens for storage and transportation of SNF and […] also […] radiation-proof ballast for the geological burial of SNF’ indicate that this concerns also speculative use of small amounts, especially because Russia has currently no active deep geological disposal programme for spent nuclear fuel (which it considers “resource” into eternity and not waste).
If all potential reuse of DU from EU sources in Russia is summarised, less than 10% will be reused in any form within the coming one or two generations. Over 90% will be passed on to the third generation and (very far) beyond for management and disposal.
This management includes (temporary) storage of UF 6 , which currently happens in Russia at environment temperatures in large open air storage places. This constitutes a risky situation, and research would be needed to get a full picture of the risks (chance and impact) of failure of containers in, for instance, surrounding forest fire situations, in which corroded containers could start leaking and the UF 6 would sublimate, causing a large HF cloud. Given the obligation of ultimate responsibility of the Member State of origin for this waste under the 2011/70/EURATOM, research would be needed into lowering this risk.
Then, Rosatom intends to convert this UF 6 to a more stable DU 3 O 8 before 2057. Although there is already quite some experience with this conversion within the EU (e.g., in France / Orano, Cadarache), the legal responsibility for this waste would oblige the EU Member State of origin, i.e. Germany, also to research the actual conversion and following of temporary storage and potential final disposal in Russia, including potential risks and risk reduction options.
In the Netherlands, DU 3 O 8 resulting from DU from the enrichment facility of URENCO in Almelo is considered waste and is stored in the VOG temporary storage at COVRA in Borssele, awaiting final disposal in a potential deep geological disposal. Because of the long half-time of U 238 , this disposal has to be virtually permanent.
U RENCO DU is waste
The production of TENORM waste in the EU delivers still poorly researched long-term issues. The fact that part of this TENORM is transferred outside of the EU only further complicates the situation, but should in essence not change the ‘ultimate responsibility’ of Euratom Member States (2011/70/EURATOM art. 4(2)) for proper handling and disposal of this radioactive material that appears as waste from industrial processes within the European Union. Especially the longevity and toxicity of the material (with a half-life of uranium-238 of 4.5 billion years) urges for research into proper disposal of this material, when it de facto will not be further used.
This is especially relevant for the exported depleted uranium from URENCO to Russia. There is currently no transparency about whether any fraction of this material is actually de facto reused, what happens with the remaining fraction in case it is reused, and which proper handling and deposition methods must be found and optimised. Also, when this material has to be considered as waste (as we argue), or certain fractions of it, repatriation of resulting wastes after processing needs to be taken into account, as well as handling methods that should be in place.
Given the fact that this DU will in Russia either be demobilised as uranium oxide before 2057 and stored for an unspecified multi-generational time, or for a tiny fraction reused under the production of radioactive wastes as by-product, we argue that the status of this from the EU to Russia exported DU should by default be that of waste.
[1] In dedication to Rashid Alimov († 2020), who delivered vital input for this chapter, but succumbed to COVID-19.
[2] See: Nikitin, Alexander, Oleg Muratov and Ksenia Vakhrusheva, Depleted Uranium Hexafluoride (current situation, safe handling and prospects), St. Petersburg (2020) Bellona Foundation; https://network.bellona.org/content/uploads/sites/3/2020/08/Depleted-Uranium-Hexafluoride.pdf – Although this publication was published under the name of Bellona, the content was provided and overseen by Rosatom
[3] Nikitin, Alexander, Oleg Muratov and Ksenia Vakhrusheva, Depleted Uranium Hexafluoride (current situation, safe handling and prospects), St. Petersburg (2020) Bellona Foundation; https://network.bellona.org/content/uploads/sites/3/2020/08/Depleted-Uranium-Hexafluoride.pdf
[4] From Nikitin (2020), page 17 – this refers to Federal Law of November 21, 1995 No. 170-FZ on use of nuclear energy.
[5] https://greenpeace.ru/wp-content/uploads/2019/10/Wirtschaftsministerium-NRW-an-GAL-Gronau-12.09.2019-UAA-Gronau.pdf
[6] https://www.wise-uranium.org/nfcue.html
[7] From Nikitin (2020), page 31.
[8] Wegener, Bernhard W., Zur Zulässigkeit von Dual-Use-Exportgenehmigungen für abgereichertes Uran von Deutschland nach Russland gemäß der EU-Verordnung 833/2014 – Rechtsgutachten für die Bundestagsfraktion Bündnis 90/Die Grünen , Erlangen (2020) Friedrich-Alexander-Universität Erlangen-Nürnberg; https://kotting-uhl.de/site/wp-content/uploads/2020/10/Gutachten-Endfassung-final.pdf
- What we do?
- Why Nuclear Transparency Watch?
- Management Board
- Legal notice
- Cookie policy
- Documentation
- European legal texts
- Aarhus Convention
- Relevant studies
- Useful documents
- Press releases
- Opinion Columns
- Press review
- Transparency and Public participation
- Nuclear Emergency Preparedness and Response
- Radioactive waste management
- Nuclear power plants
- 38, rue Saint Sabin
- 75011 PARIS
- +32 (0) 2 284 53 97

Along with Stanford news and stories, show me:
- Student information
- Faculty/Staff information
We want to provide announcements, events, leadership messages and resources that are relevant to you. Your selection is stored in a browser cookie which you can remove at any time using “Clear all personalization” below.
Nuclear reactors generate reliable supplies of electricity with limited greenhouse gas emissions. But a nuclear power plant that generates 1,000 megawatts of electric power also produces radioactive waste that must be isolated from the environment for hundreds of thousands of years. Furthermore, the cost of building a large nuclear power plant can be tens of billions of dollars.

Small modular reactors are about 1/10 to 1/4 the size of a traditional nuclear energy plant due to compact, simplified designs. (Image credit: Idaho National Laboratory)
To address these challenges, the nuclear industry is developing small modular reactors that generate less than 300 megawatts of electric power and can be assembled in factories. Industry analysts say these advanced modular designs will be cheaper and produce fewer radioactive byproducts than conventional large-scale reactors.
But a study published May 31 in Proceedings of the National Academy of Sciences has reached the opposite conclusion.
“Our results show that most small modular reactor designs will actually increase the volume of nuclear waste in need of management and disposal, by factors of 2 to 30 for the reactors in our case study,” said study lead author Lindsay Krall, a former MacArthur Postdoctoral Fellow at Stanford University’s Center for International Security and Cooperation (CISAC) . “These findings stand in sharp contrast to the cost and waste reduction benefits that advocates have claimed for advanced nuclear technologies.”
Global nuclear power
About 440 nuclear reactors operate globally , providing approximately 10 percent of the world’s electricity. In the United States , 93 nuclear reactors generate nearly a fifth of the country’s electricity supply.
Unlike power plants that run on coal or natural gas, nuclear plants emit little carbon dioxide, a major cause of global warming. Advocates say that as worldwide demand for clean energy increases, more nuclear power will be needed to minimize the effects of climate change.
But nuclear energy is not risk free. In the U.S. alone, commercial nuclear power plants have produced more than 88,000 metric tons of spent nuclear fuel, as well as substantial volumes of intermediate and low-level radioactive waste. The most highly radioactive waste, mainly spent fuel, will have to be isolated in deep-mined geologic repositories for hundreds of thousands of years. At present, the U.S. has no program to develop a geologic repository, after spending decades and billions of dollars on the Yucca Mountain site in Nevada. As a result, spent nuclear fuel is currently stored in pools or in dry casks at reactor sites, accumulating at a rate of about 2,000 metric tonnes per year.
Simple metrics
Some analysts maintain that small modular reactors will significantly reduce the mass of spent nuclear fuel generated compared to much larger, conventional nuclear reactors. But that conclusion is overly optimistic, according to Krall and her colleagues.
“Simple metrics, such as estimates of the mass of spent fuel, offer little insight into the resources that will be required to store, package, and dispose of the spent fuel and other radioactive waste,” said Krall, who is now a scientist at the Swedish Nuclear Fuel and Waste Management Company . “In fact, remarkably few studies have analyzed the management and disposal of nuclear waste streams from small modular reactors.”
Dozens of small modular reactor designs have been proposed. For this study, Krall analyzed the nuclear waste streams from three types of small modular reactors being developed by Toshiba, NuScale, and Terrestrial Energy. Each company uses a different design. Results from case studies were corroborated by theoretical calculations and a broader design survey. This three-pronged approach enabled the authors to draw powerful conclusions.
“The analysis was difficult, because none of these reactors are in operation yet,” said study co-author Rodney Ewing , the Frank Stanton Professor in Nuclear Security at Stanford and co-director of CISAC. “Also, the designs of some of the reactors are proprietary, adding additional hurdles to the research.”
Neutron leakage
Energy is produced in a nuclear reactor when a neutron splits a uranium atom in the reactor core, generating additional neutrons that go on to split other uranium atoms, creating a chain reaction. But some neutrons escape from the core – a problem called neutron leakage – and strike surrounding structural materials, such as steel and concrete. These materials become radioactive when “activated” by neutrons lost from the core.
The new study found that, because of their smaller size, small modular reactors will experience more neutron leakage than conventional reactors. This increased leakage affects the amount and composition of their waste streams.
“The more neutrons that are leaked, the greater the amount of radioactivity created by the activation process of neutrons,” Ewing said. “We found that small modular reactors will generate at least nine times more neutron-activated steel than conventional power plants. These radioactive materials have to be carefully managed prior to disposal, which will be expensive.”
The study also found that the spent nuclear fuel from small modular reactors will be discharged in greater volumes per unit energy extracted and can be far more complex than the spent fuel discharged from existing power plants.
“Some small modular reactor designs call for chemically exotic fuels and coolants that can produce difficult-to-manage wastes for disposal,” said co-author Allison Macfarlane , professor and director of the School of Public Policy and Global Affairs at the University of British Columbia. “Those exotic fuels and coolants may require costly chemical treatment prior to disposal.”
“The takeaway message for the industry and investors is that the back end of the fuel cycle may include hidden costs that must be addressed,” Macfarlane said. “It’s in the best interest of the reactor designer and the regulator to understand the waste implications of these reactors.”
Radiotoxicity
The study concludes that, overall, small modular designs are inferior to conventional reactors with respect to radioactive waste generation, management requirements, and disposal options.
One problem is long-term radiation from spent nuclear fuel. The research team estimated that after 10,000 years, the radiotoxicity of plutonium in spent fuels discharged from the three study modules would be at least 50 percent higher than the plutonium in conventional spent fuel per unit energy extracted.
Because of this high level of radiotoxicity, geologic repositories for small modular reactor wastes should be carefully chosen through a thorough siting process, the authors said.
“We shouldn’t be the ones doing this kind of study,” said Ewing. “The vendors, those who are proposing and receiving federal support to develop advanced reactors, should be concerned about the waste and conducting research that can be reviewed in the open literature.”
Rod Ewing is also a professor in the Department of Geological Sciences in the Stanford School of Earth, Energy and Environmental Sciences . The Center for International Security and Cooperation is part of the Freeman Spogli Institute for International Studies at Stanford.
This research was supported by the John D. and Catherine T. MacArthur Foundation through fellowships at George Washington University and at the Stanford Center for International Security and Cooperation.
To read all stories about Stanford science, subscribe to the biweekly Stanford Science Digest .
Media Contacts
Rod Ewing, Geological Sciences: (650) 725-8641, [email protected]
Mark Golden, Precourt Institute for Energy: (650) 724-1629, [email protected]
Lindsay Krall, Swedish Nuclear Fuel and Waste Management Company: +46 70 254 0560, [email protected]
Allison Macfarlane, School of Public Policy and Global Affairs, University of British Columbia: 617-460-1039, [email protected]
The Precourt Institute for Energy is part of the Stanford Doerr School of Sustainability .

Small modular reactors will exacerbate challenges of highly radioactive nuclear waste, new study finds
Nuclear reactors generate reliable supplies of electricity with limited greenhouse gas emissions.
But a nuclear power plant that generates 1,000 megawatts of electric power also produces radioactive waste that must be isolated from the environment for hundreds of thousands of years. Furthermore, the cost of building a large nuclear power plant can be tens of billions of dollars.

To address these challenges, the nuclear industry is developing small modular reactors that generate less than 300 megawatts of electric power and can be assembled in factories. Industry analysts say these advanced modular designs will be cheaper and produce fewer radioactive byproducts than conventional large-scale reactors.
But a new study to be published May 31 in Proceedings of the National Academy of Sciences has reached the opposite conclusion.
“Our results show that most small modular reactor designs will actually increase the volume of nuclear waste in need of management and disposal, by factors of 2 to 30 for the reactors in our case study,” said study lead author Lindsay Krall, a former MacArthur Postdoctoral Fellow at Stanford University’s Center for International Security and Cooperation (CISAC) . “These findings stand in sharp contrast to the cost and waste reduction benefits that advocates have claimed for advanced nuclear technologies.”
Global nuclear power

About 440 nuclear reactors operate globally , providing approximately 10 percent of the world’s electricity. In the United States , 93 nuclear reactors generate nearly a fifth of the country’s electricity supply.
Unlike power plants that run on coal or natural gas, nuclear plants emit little carbon dioxide, a major cause of global warming. Advocates say that as worldwide demand for clean energy increases, more nuclear power will be needed to minimize the effects of climate change.

But nuclear energy is not risk free. In the U.S. alone, commercial nuclear power plants have produced more than 88,000 metric tons of spent nuclear fuel, as well as substantial volumes of intermediate and low-level radioactive waste. The most highly radioactive waste, mainly spent fuel, will have to be isolated in deep-mined geologic repositories for hundreds of thousands of years. At present, the U.S. has no program to develop a geologic repository, after spending decades and billions of dollars on the Yucca Mountain site in Nevada. As a result, spent nuclear fuel is currently stored in pools or in dry casks at reactor sites, accumulating at a rate of about 2,000 metric tonnes per year.
Simple metrics
Some analysts maintain that small modular reactors will significantly reduce the mass of spent nuclear fuel generated compared to much larger, conventional nuclear reactors. But that conclusion is overly optimistic, according to Krall and her colleagues.
“Simple metrics, such as estimates of the mass of spent fuel, offer little insight into the resources that will be required to store, package, and dispose of the spent fuel and other radioactive waste,” said Krall, who is now a scientist at the Swedish Nuclear Fuel and Waste Management Company . “In fact, remarkably few studies have analyzed the management and disposal of nuclear waste streams from small modular reactors.”
Dozens of small modular reactor designs have been proposed. For this study, Krall analyzed the nuclear waste streams from three types of small modular reactors being developed by Toshiba, NuScale, and Terrestrial Energy. Each company uses a different design. Results from case studies were corroborated by theoretical calculations and a broader design survey. This three-pronged approach enabled the authors to draw powerful conclusions.
“The analysis was difficult, because none of these reactors are in operation yet,” said study co-author Rodney Ewing , the Frank Stanton Professor in Nuclear Security at Stanford and co-director of CISAC. “Also, the designs of some of the reactors are proprietary, adding additional hurdles to the research.”
Neutron leakage

Energy is produced in a nuclear reactor when a neutron splits a uranium atom in the reactor core, generating additional neutrons that go on to split other uranium atoms, creating a chain reaction. But some neutrons escape from the core – a problem called neutron leakage – and strike surrounding structural materials, such as steel and concrete. These materials become radioactive when “activated” by neutrons lost from the core.
The new study found that, because of their smaller size, small modular reactors will experience more neutron leakage than conventional reactors. This increased leakage affects the amount and composition of their waste streams.
“The more neutrons that are leaked, the greater the amount of radioactivity created by the activation process of neutrons,” Ewing said. “We found that small modular reactors will generate at least nine times more neutron-activated steel than conventional power plants. These radioactive materials have to be carefully managed prior to disposal, which will be expensive.”
The study also found that the spent nuclear fuel from small modular reactors will be discharged in greater volumes per unit energy extracted and can be far more complex than the spent fuel discharged from existing power plants.
“Some small modular reactor designs call for chemically exotic fuels and coolants that can produce difficult-to-manage wastes for disposal,” said co-author Allison Macfarlane , professor and director of the School of Public Policy and Global Affairs at the University of British Columbia. “Those exotic fuels and coolants may require costly chemical treatment prior to disposal.”
“The takeaway message for the industry and investors is that the back end of the fuel cycle may include hidden costs that must be addressed,” Macfarlane said. “It’s in the best interest of the reactor designer and the regulator to understand the waste implications of these reactors.”
Radiotoxicity
The study concludes that, overall, small modular designs are inferior to conventional reactors with respect to radioactive waste generation, management requirements, and disposal options.
One problem is long-term radiation from spent nuclear fuel. The research team estimated that after 10,000 years, the radiotoxicity of plutonium in spent fuels discharged from the three study modules would be at least 50 percent higher than the plutonium in conventional spent fuel per unit energy extracted.
Because of this high level of radiotoxicity, geologic repositories for small modular reactor wastes should be carefully chosen through a thorough siting process, the authors said.
“We shouldn’t be the ones doing this kind of study,” said Ewing. “The vendors, those who are proposing and receiving federal support to develop advanced reactors, should be concerned about the waste and conducting research that can be reviewed in the open literature.”
Rod Ewing is also a professor in the Department of Geological Sciences in the Stanford School of Earth, Energy and Environmental Sciences . The Center for International Security and Cooperation is part of the Freeman Spogli Institute for International Studies at Stanford.
This research was supported by the John D. and Catherine T. MacArthur Foundation through fellowships at George Washington University and at the Stanford Center for International Security & Cooperation.
Explore More

U.S. startup raises $27 million to build perovskite solar factory
Swift Solar, a Stanford spinout and a specialist in perovskite tandem photovoltaics, plans to build a factory in the U.S. in the next two to three years to manufacture thin-film solar.
- Featured Reports

William Chueh named new director of Stanford’s Precourt Institute for Energy
Chueh is a leading materials scientist and entrepreneur whose research is paving the way for better batteries and cleaner power grids.

TomKat Center for Sustainable Energy to join the Sustainability Accelerator
The move will enable TomKat's student projects to get space and funding in the Accelerator, which in turn will benefit from TomKat’s experience in entrepreneurship.
Ecotoxicology

Courtesy: US Department of Energy
December 2, 2021 / 0 comments / Reading Time: ~ 7 minutes
A Case Of Environmental Injustice: Yucca Mountain Nuclear Waste Repository
Sabrina Bacher, Joy Maria Kathrin Mangila Deller, Jaskiran K Dulai, Mariana Valdovinos-Luna
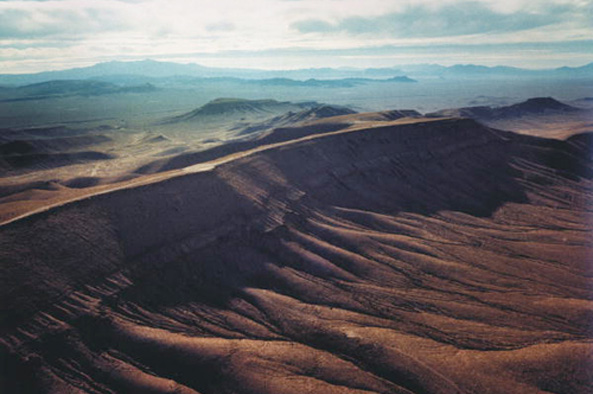
Yucca Mountain Ranges (U.S. Department of Energy)
Introductions
In 1987, the Nuclear Waste Policy Act Amendments (NWPAA) assigned Yucca Mountain, adjacent to the Nevada Test Site, as the permanent storage site for nuclear waste in the United States (Tamayo et al. 2020). The radioactive waste was stored by burying radioactive waste deep into the ground after it was completely sealed in a dry cask (Kyne and Bolin 2016).
The federal government owns approximately 85% of the lands situated in Nevada (Tamayo et al. 2020). Two areas that are highly contaminated by radioactive waste are located on American Indian-owned land. One of them is the Yucca Mountains. Two Native American tribes live close to this site among other marginalized groups: Paiute and Shoshone tribes.
This creates a problem because they do not have the financial and political capabilities to prevent this site from being permanent storage for nuclear waste (Tamayo et al. 2020). In addition to the health impacts from exposure to radioactive waste. The Native American tribes voiced out that Yucca Mountain is much more than a national sacrifice zone that the Federal government labeled, an area that holds no purpose besides storage of nuclear wastes. To the Paiutes and Shoshone tribes, Yucca Mountain gives them a sense of place to continue to have deep connections to their cultural past – a sacred land (Endres 2012).
Despite opposition from the Paiutes and Shoshone tribes, Yucca Mountain was still assigned as radioactive waste storage. During one of their attempts to communicate with the government, they were told, “We are not interested in buffalo stories” (Tamayo et al. 2020). This is a case of environmental injustice because even though they have the highest risk of exposure, they could not participate in the decision-making process. Simply because they do not have the financial or political capabilities to participate. The federal government stated that it was for the benefit of the great majority.
In an attempt to replace the previous model used for deciding where to store nuclear waste, known as Decide-Announce-Defend (DAD), that practices environmental injustice. The First National People of Color Environmental Leadership Summit stated that “environmental justice demands the right to participate as equal partners at every level of decision-making, including needs assessment, planning, implementation, enforcement, and evaluation.” Currently, it appears that there are still no permanent storage sites for radioactive waste after the operation at Yucca Mountain has been stopped.
Containment Chemistry and History, Source of Contamination
As previously mentioned, the Yucca Mountains have had a long history with radioactive waste and the presence of nuclear radiation. During the early to mid-1950s, the Nevada desert was subjected to copious amounts of radioactive weapons testing. Most notably, the infamous atomic bomb (Willett et al. 2020).
https://youtu.be/abtvODL2fn
A-Bomb Test, Yucca Flat, Nevada 05/01/1952 (Fletcher 2011)
After testing was completed, the flats remained a favorite testing space for the U.S. Military for 20 more years. Over 800 atomic and underground nuclear weapons tests took place in the 200 square miles of Yucca Valley. (Willett et al. 2020) Here, the nuclear byproducts sit in the sand and soil as radioactive isotopes of chlorine-36, uranium-235, plutonium-239, or cesium-135. Making their way into the water table and sticking around for centuries due to their long half-lives. (DOE 2009) After the military abandoned the area, many “downwinders” fell victim to the tests’ radioactive fallout. On account of the already present nature of radioactive waste, the Yucca mountains were proposed as a potential site for waste storage by the NWPPA. (DOE 2009) Researchers estimated that a storage facility would have to be designed to hold over 70,000 metric tons of radioactive waste.
Half-lives of radioactive contaminants
During the proposal period for the storage site in the early 1990s, researchers sought to determine the safety of the groundwater surrounding the facility. High levels of radioactive isotopes such as chlorine-36 were found. (Kleist 2020) Having sept through the highly porous rock layers into the groundwater and the areas proposed for tunneling. Despite the widespread protest from the local native population and ecologists alike, construction of these massive waste tunnels continued. It was only in 2010 under the administration of former President Obama that the development of over 60 miles of storage tunnels stopped. (Endres 2012)
The entrance of the 60-mile tunnels in the Yucca Valley, these massive tunnels are currently left uninhabited (Nuclear Regulatory Commission 2007)
Intersection With Environmental Justice
The nuclear waste contamination at Yucca Mountain is affecting Native communities, specifically the Shoshone and Paiute communities (Western Shoshone, 2017). As mentioned earlier, the Yucca mountain is a national sacrifice zone, and it is not just any piece of land; it is deeply rooted in the Shoshone and Paiute communities’ history (Corbin, 2004). Historically and spatially uneven politics of pollution intersect with personal and geographical imaginations. And refusing to acknowledge Aboriginal title and American Indian objections to high-level radioactive waste disposal, the US government, violates its laws. This occurs due to a confluence of historical, social, and political factors (Houston, 2012).
A copy of Native American News from 1992 detailing the fight against the Yucca Mountain Nuclear Waste Site (Broze 2019)
Western populations see these places like the wilderness and do not understand the attachment or care the native communities have with the land. As a result, they pollute and contaminate the land causing the Native communities and, in this case, the Shoshone and Paiute communities to get affected by these nuclear wastes. Places that are marked by environmental injustice are often referred to as “sacrifice zones”—areas that bear an unequal environmental burden for the greater “public good” of economic and national development (Houston, 2012).
Proposed Solutions
The capacity of nuclear waste in Yucca Mountain should not exceed its limits. Existing nuclear waste should be controlled/treated with caution to avoid creating more danger and control creating new nuclear waste.
Nuclear waste should be disposed of properly depending on its content: near-surface should be adequately locked, while geological repositories should be open (contact with the environment) or sealed (underground) (Tamayo et al. 2020).
The solution to the problem of nuclear waste remains as first proposed by the National Academy of Sciences in 1957: mined geologic repositories that isolate the waste from humans and the environment. (MacFarlane, et al. 2006) The development of disposal facilities that provide long-term isolation through the use of passive engineered and natural barriers rather than through the continued human control and maintenance. The EPA (Environmental Protection Agency) standard of 1985 set containment requirements limiting releases of radioactive species for ten thousand years, individual protection requirements limiting annual exposure (doses) for one thousand years, and groundwater protection requirements limiting radioactivity in groundwater for one thousand years (MacFarlane, et al. 2006). EPA (Environmental Protection Agency) and NRC (Nuclear Regulatory Commission) regulated the controlled area extended no more than five or ten kilometers from the primary site of waste emplacement. Later, the five to ten-kilometer boundary was enlarged to eighteen kilometers. The critical group at risk would be likely to live near Yucca Mountain and be exposed to radiation through contaminated groundwater. (MacFarlane, et al. 2006)
Takeaways

Environmental Justice (De Vries 2017).
With the long history of underrepresentation of indigenous communities, it is no surprise that the Yucca Mountain waste facility is still a possible, disposable site despite the long list of hazards associated with it. The Paiute and Shoshone tribes have been subjected to radiation contamination for decades. They are developing cancer, asthma, and reproductive problems (Houston 2012). Unfortunately, it is not as easy to educate and prevent the continuation of radioactive pollution as you can with other contaminants. Therefore this complex problem needs an even more complex solution. To help the public understand the risks associated with this contamination, the EPA should present a yearly analysis of the contaminants present in the soil around the sites and develop natural barriers to help slow the leaching of radioactive isotopes into groundwater. It should be the responsibility of the US government to help set the remedial efforts into motion as the radioactive weapons abused throughout the area were the primary source of contamination. Most importantly, however, the voices of the local communities, especially the Paiute and Shoshone tribes, must be amplified dramatically. Without the voices of these communities, they will continue to suffer in even the most basic ways.
Broze D. Trump is Trying to Revive the Yucca Mountain Nuclear Waste Site | Intercontinental Cry. [accessed 2021 Nov 29]. https://intercontinentalcry.org/trump-is-trying-to-revive-the-yucca-mountain-nuclear-waste-site/ .
Corbin A. Yucca Mountain – United States. Sacred Land. [accessed 2021 Nov 29]. https://sacredland.org/yucca-mountain-united-states/ .
Endres D. 2012. Sacred Land or National Sacrifice Zone: The Role of Values in the Yucca Mountain Participation Process. Environmental Communication. 6(3):328–345. doi: 10.1080/17524032.2012.688060 .
Houston D. 2013. Environmental Justice Storytelling: Angels and Isotopes at Yucca Mountain, Nevada. Antipode. 45(2):417–435. doi: 10.1111/j.1467-8330.2012.01006.x .
Kleist T. Yucca Mountain: Faster Water Flow Undermines Project Safety, UNR Geologist Says. [accessed 2021 Nov 29]. https://www.kunr.org/post/yucca-mountain-faster-water-flow-undermines-project-safety-unr-geologist-says .
Kyne D, Bolin B. 2016. Emerging Environmental Justice Issues in Nuclear Power and Radioactive Contamination. International journal of environmental research and public health. 13(7). doi: http://dx.doi.org/10.3390/ijerph13070700 . [accessed 2021 Nov 29]. http://www.proquest.com/docview/1805484759?pq-origsite=primo .
MacFarlane A, Ewing R, Ewing RC. 2006. Uncertainty Underground: Yucca Mountain and the Nation’s High-Level Nuclear Waste. Cambridge, UNITED STATES: MIT Press. [accessed 2021 Nov 29]. http://ebookcentral.proquest.com/lib/washington/detail.action?docID=3338498 .
Neisius M. Western Shoshone Nation Opposes Yucca Mountain Nuclear Repository – Commodities, Conflict, and Cooperation. [accessed 2021 Nov 29]. https://sites.evergreen.edu/ccc/warnuclear/shoshone-tribe-opposes-yucca-mountain-nuclear-repository/ .
Schneidmiller C. 2017. Early 2018 House Vote Expected on Yucca Mountain Bill. ExchangeMonitor. [accessed 2021 Nov 29]. https://www.exchangemonitor.com/early-2018-house-vote-expected-yucca-mountain-bill/ .
U.S. Department of Energy Office of Civilian Radioactive Waste Management. 2008 Jun. Yucca Mountain Repository License Application: Safety Analysis Report. :100.
Willett J, Tamayo A, Kern J. 2020. Understandings of environmental injustice and sustainability in marginalized communities: A qualitative inquiry in Nevada. International Journal of Social Welfare. 29(4):335–345. doi: 10.1111/ijsw.12456 .
And So It Was Written

Author: Sabrina Bacher, Joy Maria Kathrin Mangila Deller, Jaskiran K Dulai, Mariana Valdovinos-Luna
Published: December 2, 2021
Word Count: 1628
Reading time: ~ 7 minutes
ORGANIZED BY
More to read, provide feedback cancel reply.
Your email address will not be published. Required fields are marked *
Save my name, email, and website in this browser for the next time I comment.
A TRU Writer powered SPLOT : Ecotoxicology
Blame @cogdog — Up ↑

Low-Level Radioactive Waste Management and Disposition: Proceedings of a Workshop (2017)
Chapter: 3 successful disposition case studies, successful disposition case studies.
Rebecca Robbins, planning committee member and predisposal unit head at the International Atomic Energy Agency (IAEA), moderated this session, which used case studies to highlight examples of successful low-level waste (LLW) management and disposal within current regulatory frameworks. The case studies presented situations in which previously challenging LLW streams 1 were successfully managed and disposed of. The first two presentations in this session provided case studies from the United States; the next two presentations focused on case studies from outside the United States. A discussion was held after all of these case studies had been presented.
The comments from the moderators, the panelists, and other workshop participants are their own. They do not necessarily represent official views of their employers, governments, or other organizations that may be mentioned in the presentations or discussions.
Dr. Robbins began the session by requesting the workshop participants, as they listened to each case study, to identify the “key characteristics” that contributed to its success. Key characteristics include the practices, activities, attitudes, and actions with respect to the case studies and associated regulatory frameworks.
Melanie Pearson Hurley, headquarters liaison in the Office of Field Operations within the Department of Energy (DOE), presented a DOE case study. Greg Lovato, deputy administrator at the Nevada Division of
___________________
1 “Challenging LLW streams” are defined as LLW streams that have potentially non-optimal or unclear disposition pathways due to their origin or content and incompatibility with existing standards, orders, or regulations.
Environmental Protection (NDEP), provided examples of key characteristics for successful disposition from the perspective of a state regulator. For international case studies, Miklos (Mike) Garamszeghy, design authority and manager of technology assessment and planning for the Canadian Nuclear Waste Management Organization, provided two examples from Canada and Gérald Ouzounian, international director for the National Radioactive Waste Management Agency (ANDRA), provided a case study from France.
3.1 UNITED STATES CASE STUDIES
Case study 1: separations process research unit tank waste sludge.
Mrs. Hurley presented the Separations Process Research Unit (SPRU) project as DOE’s case study. In the early 1950s, research on plutonium and uranium separation techniques such as PUREX and REDOX 2 was performed at SPRU within the Knolls Atomic Power Laboratory (KAPL). 3 KAPL, now an active naval nuclear laboratory, is located near Schenectady, New York, adjacent to the Mohawk River. The inactive SPRU facilities occupy about 5 acres of land immediately adjacent to KAPL.
The research at SPRU was performed on a laboratory scale and supported larger operations at both the Hanford Site in Washington and the Savannah River Site in South Carolina. Radioactive liquid and sludge wastes resulting from the SPRU research were stored in seven tanks located on site. The SPRU project timeline was established by the demolition dates for the buildings in which the research was performed and the wastes were stored. There was a strict requirement that the sludge waste be removed and disposed of by spring 2014.
Figure 3-1 provides a cross-section and plan view of two facilities at SPRU. The top drawing is a cross-section of the G2 building, which housed the laboratories, hot cells, and separations processing and testing equipment, and the H2 building, which was used for liquid and solid waste processing. The G2 and H2 buildings are connected by an underground tunnel. The lower drawing in Figure 3-1 shows the plan view of buildings G2 and H2. The tank farm in the lower-right corner of the figure is the focus of this presentation.
The radioactive waste from chemical processing was stored in the H2 tank farm (seven underground concrete-enclosed stainless steel tanks). This waste included about 200 cubic feet (5.7 cubic meters) of sludge consisting
2 REDOX (reduction oxidation) and PUREX (Plutonium and Uranium Recovery by Extraction) are processes for separating uranium and/or plutonium from irradiated fuel and targets.
3 In the 1950s, KAPL was a government research laboratory created by the U.S. Atomic Energy Commission (a predecessor agency to DOE).

of fine particulates and liquids containing fission products, mostly cesium and strontium, and long-lived transuranic (TRU) radionuclides, primarily plutonium-239. The sludge contained 36 curies of total radionuclides, including 2.5 to 6.5 curies of TRU radionuclides. The concentration of the long-lived TRU radionuclides in the final waste packages ranged from 11.5 to 65.5 nanocuries per gram (nCi/g).
The total mercury content of the sludge was more than 1 percent, and it contained high levels of lead, chromium, and cadmium. This led to an initial determination that the sludge may be a Resource Conservation and Recovery Act (RCRA) characteristic hazardous waste 4 for metals. This waste classification would have complicated the management of the sludge because the hazardous component would be regulated by the Environmental Protection Agency (EPA) in addition to DOE’s regulation of the radioactive component. However, two toxicity characteristic leaching pro-
4 “EPA: Defining Hazardous Waste: Listed, Characteristic and Mixed Radiological Wastes,” accessed February 25, 2017, https://www.epa.gov/hw/defining-hazardous-waste-listedcharacteristic-and-mixed-radiological-wastes#character .
cedures (TCLP) 5 confirmed that the hazardous component of the waste was only at 0-3 percent of regulatory levels, due to the low solubility of the metals in the sludge. Consequently, the sludge was determined to not contain hazardous waste and could more simply be managed under DOE orders.
DOE Order 435.1, Radioactive Waste Management , was used to guide decisions on disposing of the sludge from SPRU. The Order allows for the disposition of LLW in federal or commercial facilities. An exemption request must be approved by DOE headquarters for waste to be disposed of in a commercial disposal facility. Approval will be given if commercial disposal demonstrates compliance with regulations and waste acceptance criteria (WAC), is cost-effective, and is determined to be “in the best interests of the United States government.”
There were two disposal options for the SPRU sludge: the Nevada Nuclear Security Site (NNSS), a DOE disposal site in Nevada, and Waste Control Specialists (WCS), a commercial disposal site in Texas. Both disposal options were explored, and WCS was selected, in part due to the compressed schedule 6 for completing cleanup of the SPRU tanks (spring 2014).
DOE worked closely with Texas regulators and WCS on establishing the waste profile 7 through the standard process described in the WCS Waste Acceptance Plan. 8 Texas regulators accepted DOE’s policy that waste is not formally classified until all processing is completed and a stabilized waste form is produced. Mrs. Hurley identified this close collaboration as a “key characteristic” for successful disposition of the sludge.
The plan was to have the waste stabilized using a mixture of cement, fly ash, and slag that was then solidified in the final waste package for transportation and final disposal. The sludge solidification system at SPRU was designed and cold tested off site by the vendor and then installed in the H2 tank vault area. Cold-test operations were conducted on site prior to hot operations to ensure the system would perform as designed.
Figure 3-2 is a schematic of the H2 tank vault area and processing systems. Mrs. Hurley noted that there was an airborne release of radioac-
5 TCLP testing determines the mobility of organic and inorganic chemical species within in liquid, solid, and multiphasic wastes. TCLP testing follows specific guidelines established by EPA.
6 DOE had an existing contract with WCS, and WCS allowed for a shorter waste profile review time.
7 “Waste profiles” are required documents for shipping and acceptance of waste. The waste generator must submit a waste profile of each waste package for approval by the disposal facility prior to shipment. The disposal facility reviews the waste profiles to confirm the waste is compliant with the WAC of the disposal site.
8 “Application for License to Authorize Near Surface Disposal of Low-level Radioactive Waste, Appendix 5.2-1: Waste Acceptance Plan Revision 9,” see Section 5.2: Waste Profile Approval, accessed February 25, 2017, http://www.wcstexas.com/wp-content/uploads/2016/01/Waste-Acceptance-Plan.pdf .

tive material at SPRU in 2010. As a consequence of this event, the EPA required DOE to construct a tent enclosure over the H2 facility with portable ventilation units (contained in the outer tent, Area H2 Tent, shown in Figure 3-2 ). Underneath this larger tent is another tent (Existing Big Top Tent in Figure 3-2 ) that originally served as a weather enclosure over the tank farm. This weather-enclosure tent was retained when the larger enclosure was constructed to add another level of protection.
Within the Big Top Tent are two additional tents, the Tank Containment Retrieval and Solidification Containment Tents (see Figure 3-2 ). The sludge retrieval, mixing, processing, and characterization operations were carried out in these tents. Batches of sludge were retrieved from the 509E Tank, 9 mixed to suspend the solids in the waste, transferred to the final waste package, and then combined with cement, fly ash, and slag. The mixture was periodically checked by a penetration test to determine when it was solidified. If there was any remaining free water, additional cement mix was added.
The waste package was moved into a shielded temporary storage area set up in the G2 building ( Figure 3-1 ). 10 The cement mixture curing times were long because the storage area was unheated. Once fully cured, the waste packages were shipped to WCS for disposal.
Sludge processing began on September 9, 2013, and the final shipments to WCS were completed on February 27, 2014. 11 Nearly 10,000 gallons of sludge were processed and solidified in 28 liners. The liners were shipped to WCS via trucks. (There were two liners per truckload and a total of 14 truck shipments.) This campaign removed the majority of the radionuclides from the SPRU site and allowed DOE’s deactivation activities to continue in the H2 basement as scheduled.
While this case study highlights many successes, there were obstacles to overcome, including the following:
- Working within a decades-old facility with limited physical and onsite storage. There was no lay-down area where more than one liner could cure at the same time, and the temporary storage area in the G2 building allowed for 3 to 4 liners at a maximum.
- Retrieving sludge from the 509E Tank, including cleaning out solids near the bottom of the tank.
- Working with a waste stream (sludge) that is difficult to characterize and process. A continuous mixing system was used to keep
9 In 2010, the sludge was consolidated into a single tank, the 509E tank, in preparation for waste processing and disposition.
10 Mrs. Hurley noted that, at the same time the liners were temporarily stored there, deactivation activities were also taking place to prepare for demolition of the G2 building.
11 The schedule accounted for the fact that concrete would not fully cure during the winter months (the SPRU tanks were covered by an unheated processing tent).
solids suspended in the waste so that the final waste form was homogenous.
- Performing the sludge processing work immediately adjacent (less than 25 feet or 7.6 meters) to a currently operating research and development laboratory and during deconstruction of the G2 building.
- Performing this work in a tent-type containment structure ( Figure 3-2 ). Portable ventilation units and the HEPA 12 filters were used to ensure that safe working conditions were maintained.
- Addressing waste classification uncertainties. DOE performed historical research and additional evaluations to show that the sludge waste was not high-level waste and could be managed as LLW.
Several key management practices contributed to the success of this project:
- A dedicated and technically competent workforce that understood the mission objective and the importance of safety, including an excellent DOE federal project director.
- Frequent communications among the DOE participants, DOE staff from headquarters, NNSS, DOE’s consolidated business center in Cincinnati, and KAPL, the adjacent research and development laboratory. Support from a “Senior Integrated Project Team” was also key to the success of the project.
- Cold testing of the treatment system at the vendor site and on site prior to operation enabled the right combination of nozzles, sluicing, and camera angles to confirm that the solids were removed from the 509E Tank.
- Early and frequent communication and engagement with the waste disposal experts from WCS.
- Coordination with the expertise throughout the DOE complex on packaging and transportation.
A participant asked Mrs. Hurley how DOE verified that solidification was adequate during cold testing. She responded that the cold testing was primarily to confirm the pump’s ability to mix the solids and liquids and to confirm homogeneous mixing. Solidification was not tested or verified during cold testing; rather, a cement and fly ash “recipe” that was used successfully at other sites was used to solidify the SPRU sludge.
12 HEPA is the acronym for high-efficiency particulate air.
Case Study 2: Low-Level Radioactive Waste Streams Reviewed for Disposal at the NNSS: Key Characteristics, Variation, and Management
Mr. Lovato’s presentation included an overview of the waste disposal sites at the NNSS, the waste profile review process, key waste stream characteristics and their variation, and key management steps taken to address some of those different characteristics.
Mr. Lovato explained that NDEP was participating in the workshop because of a memorandum of understanding between the governor of Nevada and the secretary of DOE. One of the goals of the agreement is to hold a workshop to bring more transparency and predictability to DOE’s waste disposal decisions. Mr. Lovato expressed thanks that the workshop was taking place. He noted the desire by Nevada citizens for context and predictability in DOE disposal decisions and asked the workshop participants for help in developing a LLW classification system that would foster greater confidence in future disposal decisions; he also admitted that these requests were tall orders.
Mr. Lovato suggested one way to think about Nevada’s participation in this workshop is illustrated by a famous line from the movie Jerry Maguire , in which the sports agent, played by Tom Cruise, is trying to negotiate a contract for a professional athlete, played by Cuba Gooding, Jr. The sports agent repeatedly asks the athlete to “Help me, help you.” The goal of the memorandum of understanding between Nevada and DOE is to “Help us, help you.”
The NNSS is located about 65 miles northwest of Las Vegas. The Area 5 disposal facility is a secure, 740-acre site located in the southeast corner of the NNSS (see Figure 3-3 ). The disposal facility is used to dispose of mixed LLW 13 under a RCRA permit with the state of Nevada. The waste is disposed at depths of up to 24 feet (7.3 meters).
Area 5 receives less than 5 inches (13 centimeters) of annual rainfall, and depth to groundwater is 770 feet (235 meters). Infiltration of precipitation below the plant root zone ceased between 10,000 and 15,000 years ago. Consequently, migration of the waste to groundwater is less of a risk than surface erosion from thunderstorms.
NNSS accepts approximately 1.0-1.5 million cubic feet (28,000-43,000 cubic meters) of LLW, mixed LLW, and classified waste 14 per year from more than 25 different DOE facilities. This amounts to between 5 and 10
13 LLW containing hazardous chemicals is referred to as “mixed LLW.”
14 DOE defines “classified waste” in Order 435.1 as ( DOE, 1999 , p. I-2): “Radioactive waste to which access has been limited for national security reasons and cannot be declassified shall be managed in accordance with the requirements of DOE 5632.1C, Protection and Control of Safeguards and Security Interests , and DOE 5633.3B, Control and Accountability of Nuclear Materials .”

percent of the volume of wastes disposed of across the DOE complex, including DOE wastes disposed of at commercial disposal sites ( Marcinowski, 2016 ).
NDEP is a member of the Waste Profile Review Team. The team includes DOE, contractors, and three members of NDEP and meets weekly to review waste profiles against the NNSS WAC. If a waste stream does not meet the WAC, it will not necessarily be rejected for disposal at the NNSS. The performance assessment for the facility can be reanalyzed to determine whether the waste stream under consideration would meet the facility’s performance objectives.
LLW can have a broad spectrum of characteristics. Table 3-1 provides a list of key characteristics of the LLW and mixed-waste streams considered for disposal at the NNSS. (This list was developed by Mr. Lovato based on his experiences at the NNSS.) The table shows that these waste streams have a wide range of half-lives, activities (expressed as a ratio to WAC thresholds), and plutonium equivalent grams.
Using a “plutonium equivalent grams” (PE-g) is a way to normalize the activity of different isotopes in a single package to a single standard (the activity of plutonium-239). This normalization allows for the easy determination of whether a package meets the WAC for the NNSS. (The WAC specifies a PE-g limit for each package.) The WAC for the Waste Isolation Pilot Plant (WIPP) also contains a plutonium equivalency criterion. The list of radionuclides in the WAC for the NNSS is far longer than that for WIPP, suggesting that the NNSS deals with a more diverse range of waste streams. In fact, waste characteristics at the NNSS can have a 6-17 order-of-magnitude range in values (see Table 3-1 ).
Waste management decisions are usually handled on a case-by-case basis to ensure that waste streams are appropriate for disposal at the NNSS and that stakeholder concerns are addressed. Some of the management steps used at the NNSS include decisions to adjust burial depth or transportation routing, conducting exercises in outreach and notification, and ensuring conditions on any waste profile approvals are met.
Case-by-case decisions can seem ad hoc, subjective, and reactive with-
TABLE 3-1 Variation of Key Characteristics in NNSS LLW Profiles.
Radionuclide Half-Life (years) | Ratio of Waste Isotope Activity Level to WAC Thresholds (unitless) | Plutonium Equivalent Grams (g/m ) | ||
---|---|---|---|---|
5 to 700,000, | 000 | 10 to 2 × 10 | 2.1 to 3,000,000 |
SOURCE: Modified from G. Lovato, Nevada Division of Environmental Protection.
out a reference system to compare the decisions to—especially when viewed from the outside. Nevada is interested in facilitating alternatives to disposal at the NNSS, for example by the preventing waste streams from being created and finding alternative disposal locations.
Mr. Lovato suggested a potential categorization scheme for LLW that could aid in final disposition decisions ( Table 3-2 ). This scheme proposes a few key physical, chemical, and radiological characteristics and hazards of LLW that should be considered for its safe and secure management and disposal. Also included are key characteristics of a disposal site (i.e., location, security, and control options such as inherent and engineered barriers of a site). A new regulatory framework would break down these characteristics based on the variety of potential LLW streams and transparently list the proposed disposal criteria.
Mr. Lovato suggested that the regulatory framework should be scalable when considering new LLW streams: concerns about the new LLW stream from the waste generators, recipients, public, and DOE should be captured; options for addressing those concerns should be identified using characteristics similar to those in Table 3-2 ; and options for the management and disposal of a new LLW stream should be compared against each other in a transparent way. The idea is that this new framework could be created a priori without having knowledge of the LLW streams. This type of regulatory framework would be helpful in providing context on LLW disposal decisions.
Mr. Lovato encouraged the participants not to lose heart in terms of trying to develop a better LLW categorization scheme. He acknowledged that past LLW disposal decisions were likely made for expediency and were weighed against what disposal options and regulatory frameworks were available at the time. But it is incumbent upon us in the present day to improve the system, so that future stakeholders have much-needed context for the decision-making process, which may ultimately improve stakeholder confidence in LLW management and disposal decisions.
TABLE 3-2 Potential Categorization Scheme of LLW to Guide Disposition Decisions
Half-Life Activity | Where? (Transport?) | Long Term Protection Radiation Exposure | What control options should be evaluated? |
Fissile Content PE-g | (Disposal?) | Nuclear Criticality Security | What criteria should be examined? |
Surface Dose | |||
Leachability |
SOURCE: Nevada Division of Environmental Protection.
Dr. Robbins asked a clarifying question related to Nevada’s desire to facilitate alternatives to the creation of waste streams. Was there a particular waste stream that does not fall within the NNSS’ remit to accept? If so, can the NNSS discuss the possible acceptance of this waste stream with the waste generator?
Mr. Lovato explained that it is important to the NNSS and Nevada to not only look for alternative disposal options, but also alternative technologies for generating wastes. For example, the NNSS is seen as the disposal facility for sealed sources. But in Nevada’s view, disposal of sealed sources should not default to a single location. So, Nevada is considering alternatives, such as reducing the use of sealed sources to begin with or by considering alternative disposal pathways, so that the NNSS is not relied on for disposal of all sealed sources.
3.2 INTERNATIONAL CASE STUDIES
Case studies 3-4: two low-level waste case studies from canada.
Mr. Garamszeghy’s presentation was split into three parts: background on Canadian nuclear regulation and management, a case study on the Port Hope Area Initiative (PHAI), and a case study on the Deep Geological Repository for low- and intermediate-level wastes. The PHAI disposal facility is currently under construction. The Deep Geological Repository facility for low- and intermediate-level wastes is still in the regulatory approvals phase.
There are 19 operational power reactors at four sites in Canada (three sites in Ontario and one in New Brunswick). All are CANDU 15 pressurized heavy water reactors, and all are owned by the provincially owned electric utilities (Ontario Power Generation [OPG] and New Brunswick Power). Eight of the reactors in Ontario are leased to a private firm for operation, but OPG retains the responsibility for the waste produced by these reactors and for their decommissioning. There are seven other power reactors in Canada in different stages of decommissioning. There are also seven research reactors in Canada, two reactors (one operating, the other shut down) at the Canadian Nuclear Laboratories (located near Chalk River, Ontario) and the others at universities. 16 There are numerous other historic and legacy sites undergoing decommissioning or remediation.
The Canadian Nuclear Safety Commission (CNSC) is the federal nuclear regulator, equivalent to the U.S. Nuclear Regulatory Commission
15 CANDU refers to CANada Deuterium Uranium reactors. For more information, see: “Canadian Nuclear Association: CANDU Technology,” accessed February 25, 2017, https://cna.ca/technology/energy/candu-technology/ .
16 “Canadian Nuclear Association: Research Reactors,” accessed February 25, 2017, https://cna.ca/technology/research-development/research-reactors/ .
(USNRC) in the United States. Unlike Agreement States in the United States, the CNSC has not devolved any regulatory responsibilities to Canadian provinces. 17 The Canadian Environmental Assessment Agency (CEAA) is the federal agency responsible for the environmental assessment process. In the past there was a Joint Review Panel, which was a project-specific panel set up jointly by the CNSC and the CEAA, to review environmental assessment applications and specific license applications. This process is no longer used for nuclear projects. The proponent or the project owner/operator also has responsibilities as the eventual license holder. The proponents prepare the environmental assessment, the safety report, and the thousands of pages of support documentation.
The CNSC takes its authority from the Nuclear Safety and Control Act of 2000. It is a “quasi-judicial administration tribunal” that reports directly to Parliament. The commission members are independent and mostly part-time. All of the commission hearings are open to the public and are webcasted.
The CNSC has federal jurisdiction over both nuclear facilities and activities, much the same as the USNRC. It also provides regulatory oversight of all the licensees and disseminates objective scientific, technical, and regulatory information to the public—a fairly important role when it comes to public engagement for nuclear- and waste-related projects. The decisions of the CNSC can only be challenged through judicial review in federal court. The CNSC’s decision making is transparent and science-based, at least in theory.
Risk assessments that apply to waste disposal include both a normal evolution scenario (climate change and gradual loss of engineered barriers) and disruptive scenarios (such as human intrusion). The assessment timeframe encompasses the time of maximum calculated impact (e.g., peak dose). In the case of a radioactive waste disposal facility, that time may be several million years in the future. The dose constraint for the normal evolution scenario is 0.3 milliseiverts per year (mSv/yr), equivalent to 30 millirem per year (mrem/yr). For disruptive scenarios, it is usually only a guideline of 1 mSv/yr (or 100 mrem/yr).
Canada has several types challenging LLW streams including:
- — significant amounts of carbon-14 from CANDU reactors,
- — irradiated/activated zirconium and niobium hardware from reactor refurbishments,
- — high-activity cobalt-60 waste, and
17 Mr. Garamszeghy identified one exception as some uranium mines in Saskatchewan, which has a dual federal-provincial regulatory framework.
- — stored tritium (each storage canister holds about half a million curies of tritium).
- Waste from small waste generators who may have difficulty identifying disposal pathways, especially for intermediate-level waste; and
- Large volumes of historic wastes, of which characteristics and quantities not always well documented.
The PHAI will dispose of approximately 2 million cubic meters of waste, mostly soils, in engineered mound-type facilities with multicomponent caps. This disposal will take place in two locations near Port Hope and Port Granby, located east of Toronto. The Port Hope facility is expected to be in operation in 2017; the Port Granby facility is expected to be in operation in 2018. Most of the wastes to be disposed of in these facilities are located at these facilities or nearby.
The history of the sites that are hosting these facilities can be seen in Box 3-1 . The Port Hope site was used first for radium refining and later for uranium refining. These activities contaminated the site and produced large volumes of waste. A task force was established in 1988 to find a site in Canada to dispose of the Port Hope wastes. The task force was unable to reach an agreement with a community in Canada to host a site primarily because of concerns about transporting large volumes of radioactive waste.
In 1997, Hope Township initiated a proposal to construct a long-term waste management facility near the Port Hope site. The PHAI was initiated in 2001, and environmental assessments were completed for Port Hope and Port Granby projects by 2009. Part of the agreement includes the Property Value Protection (PVP) program, which will compensate homeowners should the value of their property be reduced by the presence of the facilities.
The CNSC granted the construction license for the facility in Port Hope in 2009 and a construction license for Port Granby in 2011. The federal government made a major commitment of more than $1 Canadian billion to fund the construction of the two sites in 2012.
The Deep Geological Repository for low- and intermediate-level waste will be used to dispose of OPG-owned waste (i.e., waste from the operation and maintenance of OPG-owned facilities). The repository site is located near the Bruce Nuclear Generating Station on the eastern shore of Lake Huron in Ontario.
The community near the Bruce station volunteered to host the disposal facility. The community preferred that a single facility be used to dispose of all of OPG’s waste. Accordingly, a deep geologic repository was designed for co-disposal of low- and intermediate-level wastes. A near-surface facility would not have been able to accept all of the intermediate-level wastes
currently stored on the site. Also, a single deep geologic repository is less costly than building two separate disposal facilities.
The repository has a design capacity of about 200,000 cubic meters as packaged for disposal at a reference depth of 680 meters. Operation was originally expected to begin in the mid-2020s. The repository is currently in the regulatory review process (which is taking longer than the originally scheduled 2 years).
The official hosting agreement was signed in 2004 and was approved by the community in 2005 based on an independent poll of all year-round and seasonal residents. 18 It provides approximately $30 million in compensation to both the official host town (Kincardine) and other surrounding communities. The compensation is tied to project milestones until the repository construction is complete. After disposal operations begin, the compensation is akin to an annual fee.
The environmental assessment and licensing documentation was submitted to the CSNC in April 2011, but Canadian federal elections delayed the appointment of the Joint Review Panel until January 2012. The Joint Review Panel then implemented a public comment period that was originally planned to last for 90 days. However, the period was repeatedly extended and lasted for more than 1 year. There were, in total, 31 days of public hearings, which created 20,000 pages of documentation and more information requests from the Joint Review Panel and public. The Panel’s report was submitted to the CSNC in May 2015; it strongly recommended the repository proceed to the licensing phase.
CEAA then held a public comment period. A decision by the Minister of Environment was expected in September 2015 but was subsequently extended to December. Another Canadian federal election in fall 2015 resulted in a change in government. The new minister asked for more work to be done. The responses to the minister’s request are expected to be submitted by the end of 2016 with a final decision by the minister on the environmental assessment in early 2017. 19 If the minister approves the project it will move to the licensing phase.
This project has had several successes. Throughout the public review—with extensive local, national, and international scrutiny—the scientific evidence remained sound and passed all credible challenges. Despite a number of changes in government, local leadership, and residents, the politicians
18 There is a large contingent of weekend cottage owners in the area. When the poll was conducted, both full-time and part-time homeowners were contacted.
19 Note: the most recent update on this process was posted on April 15, 2017. The public comment period was closed on March 7, 2017. On April 5, 2017, CEAA requested additional information from OPG. “CEAA: Deep Geologic Repository Project for Low and Intermediate Level Radioactive Waste,” accessed April 27, 2017, http://www.ceaa-acee.gc.ca/050/detailseng.cfm?evaluation=17520 .
and the local community remained supportive. The project delays have allowed some opposition groups in Canada and the United States to organize and gain some support. Some members of the public became confused between two nuclear waste disposal projects planned in the same area, one for OPG’s low- and intermediate-level waste and the other for spent fuel. Public outreach continues, and OPG continues to respond to public questions and concerns. The formal decision by the Minister will define the project’s next step.
Case Study 5: The French Case: Low-Level Radioactive Waste Management
Dr. Ouzounian’s case study provided insight into the French approach to disposing of very low-level waste and LLW. He noted that his presentation focused mostly on the LLW because it is more challenging and more interesting in terms of approach and process.
ANDRA is responsible for the long-term management of all radioactive waste produced in France. The agency is independent from waste producers and reports to ministers in charge of the environment, energy, and research. It has approximately 650 employees with an annual budget of €250 million. ANDRA’s work is performed within the framework of the Planning Act of June 28, 2006 on the sustainable management of radioactive materials and wastes. 20
Safety of the population and protection of the environment are set by a national framework law and are of the highest priority in determining disposal pathways for waste. Forecasts and inventories of waste lead to a National Management Plan, which is used to identify disposition pathways for all types of waste.
There is an effort to identify a safe disposition pathway proportionate to the hazard for each type of waste. French regulations do not allow for clearance of wastes from nuclear-related activities. France uses a policy of “waste zoning” at the generator’s plant to segregate waste from zones that generate radioactive waste from those that do not.
The French radioactive waste classification scheme is shown in Figure 3-4 and described below:
- Intermediate-level and low-level wastes are generated by the day-to-day operations at the nuclear power plants (NPP; green box in Figure 3-4 ). These wastes, previously disposed of at the Centre de la Manche disposal facility (CSM), are currently being sent to the
20 “ANDRA: Overview of national policy concerning radioactive waste management,” accessed February 25, 2017, http://www.andra.fr/international/pages/en/menu21/national-framework/overview-of-national-policy-1593.html .

Centres de stockage de l’Aube (CSA), which has been operational since 1992.
- Intermediate-level and high-level wastes are generated during uranium fuel recycling (i.e., reprocessing) (pink box in Figure 3-4 ). This waste will be stored in the geological disposal facility, the Cigéo Project. 21
- Very low-level waste is generated from shut-down and decommissioning (or dismantling) operations. This waste is disposed of at the Centre Industriel de Regroupement, d’Entreposage et de Stockage (CIRES) facility (upper blue box in Figure 3-4 ).
- Low-level, but long-lived, waste, is generated from graphite gas-cooled reactors and, for example, from the production of rare earth metals (lower solid blue box in Figure 3-4 ).
21 France has made progress toward addressing its intermediate- and high-level wastes through the Cigéo Project, constructed in a clay formation at 500 meters depth and expected to be commissioned by 2025.
Waste from small producers or other nuclear activities can span the range of waste types shown in Figure 3-4 but represents a minor part of the inventory.
There are two characteristics shown in Figure 3-4 : activity levels and half-lives. Activity levels (rows in Figure 3-4 ) span orders of magnitude (less than 100 becquerels per gram [Bq/g] to more than 1 billion Bq/g) because there are specific threshold values for each radionuclide. Activity levels for very low-level waste range from 0 to 100 Bq/g with an average value of approximately 10 Bq/g. Waste is classified as “short-lived” or “long-lived” based on whether its half-life is less than or equal to or greater than 31 years, respectively (columns in Figure 3-4 ). The 31-year half-life is approximately the half-life of cesium-137, which is 30.17 years. 22
It is not possible from an operational standpoint to separate short-lived and long-lived radionuclides in NPP waste. There are always some long-lived radionuclides in this waste. WAC for very low-level and low-level disposal facilities in France allow for the disposal of waste containing certain amounts of long-lived radionuclides.
The principles behind radioactive waste disposal in France are, first, to contain and isolate the waste until it reaches a level of activity that does not represent significant hazard to the public or the environment (the monitoring phase in Figure 3-5 ). And, second, to limit the transfer of waste to the biosphere and to humans (the post-monitoring phase in Figure 3-5 ). As seen in Figure 3-5 , the containment phase lasts for about 300 years for near-surface disposal of waste with low levels of activity and several hundreds of thousands of years for geological disposal of high-level waste.
Dr. Ouzounian described the CSA disposal facility for low-level and intermediate-level short-lived waste. The facility was licensed and commissioned in 1992 with a total capacity of 1 million cubic meters—enough capacity to contain all of the low- and intermediate-level radioactive waste generated by the present fleet of French NPPs (58 reactors). The CSA facility was designed to contain and isolate the waste for 300 years, as required by the monitoring requirement mentioned previously, and to meet the requirements for the long-term post-monitoring phase.
The French waste disposal system employs the “defense-in-depth” concept with a multi-barrier system. The system consists of the waste package, which includes a containment material enveloping the waste (the first barrier); the disposal vault, which includes a network control gallery to control water that may flow through the disposal facility and final cover (the second barrier); and the geological environment, which has natural barriers such as
22 The Planning Act of June 28, 2006 on the sustainable management of radioactive materials and waste specifies that the half-life cut-off between short-lived and long-lived waste is 31 years.

clay to retard waste migration (the third barrier). This third barrier is the most important barrier in the post-monitoring phase.
Figure 3-6 is a schematic of the defense-in-depth disposal concept. A draining layer underlays the disposal facility, which in turn is underlain by an impermeable layer. The water table is shown with an outlet, labeled as “source” in the figure.
Inventory monitoring is essential for the effective management of radioactive waste—especially for managing long-lived radionuclides such as carbon-14, chlorine-36, and some beta emitters. NPP operators do not generally monitor for these isotopes because they do not impact daily plant operations. Therefore, the French regulator has established specific characterization requirements for these radionuclides for disposal purposes. For near-surface waste disposal, long-lived radionuclides are the major contributors to public doses in the post-monitoring phase.
Dr. Ouzounian’s presentation also introduced France’s approach to safety assessments, details on waste control acceptance criteria, and examples highlighting key aspects of safe operations and the defense-in-depth concept. Of particular relevance to this workshop was a discussion on the WAC for waste packages. These include:
- Radiological content
- Physical characteristics
- Chemical stability
- Gas generation
- Expected performance for long time periods
- Leaching rate
- Uniform distribution within the waste package (no hot spots)
Dr. Ouzounian provided historical perspective on the progression of safety rules, disposal concepts, and protection criteria in France. The safety rules were defined progressively, learning through the operational experiences of disposal facilities. Documents were updated and improved according to the experience of the operators—not the regulatory body. However, any changes to improve the safety rules are validated and endorsed by the regulatory body. General operational rules, and safety and radiation protection criteria, are also updated continuously.
John Applegate, the planning committee chair and executive vice president for University Academic Affairs of Indiana University, asked where the WAC (bulleted list above) came from and whether they had a risk basis. Dr. Ouzounian noted that the WAC were generated from safety assessments. Mr. Applegate also commented that experience at the prior disposal facility (CSM) appeared to be very helpful in designing the new facility (CSA), to which Dr. Ouzounian strongly agreed. All the incidents and malfunctions
that occurred with the first disposal facility—which was designed without the benefit of detailed computer models—allowed for improvements to the new facility. The first safety regulations (1984 and 1985) are the result of the experiences from the first facility.
Dr. Ouzounian also noted the importance of adapting to knowledge gained from waste disposal experience in general. The process of developing an approach for the management and disposition of nuclear waste began in 1969, and much has been learned progressively. For example, it is now clear that the physical processes likely to occur should be well-understood and well-described, which requires high-quality modeling due to the long timescales involved. It is not possible to run an experiment for 100 to 300 years (or longer) to determine what may happen. The values, characteristics, and sources of hazards that are used in our assessments are the result of the models. This is why waste disposition decisions are site-specific, and also why we cannot transpose from one site to the other.
Dr. Robbins asked for clarification on one aspect of the French waste classification scheme. Is the irradiated graphite shown in Figure 3-4 considered LLW or intermediate-level waste according to the French classification scheme? Dr. Ouzounian explained that it is considered to be low-level but long-lived radioactive waste. One of the disposal options being studied is to segregate different types of graphite for disposal in different types of facilities depending on its irradiation level and activity.
3.3 DISCUSSION: KEY CHARACTERISTICS OF LLW AND CHALLENGING LLW STREAMS
Workshop chair John Applegate moderated the closing discussion on the first day’s presentations. He noted that three organizing elements for managing challenging LLW streams were discussed:
- Characteristics of the waste. Defining waste characteristics is a technical issue. Mr. Applegate suggested that one could identify which characteristics are most important for making LLW disposal decisions. Alternatively, one could identify which characteristics are not important and are unnecessarily complicating waste disposal decisions.
- Waste management practices. Mr. Applegate asked whether participants could identify management practices that were unnecessarily slowing waste management decisions.
- Regulatory framework. Mr. Applegate asked participants to identify aspects of the current U.S. regulatory framework that are perceived to be failing. What can we learn from the experiences of other nations and international bodies? Mr. Applegate noted that
regulatory flexibility is seen to be both useful as well as problematic. How do we manage that flexibility to make it useful, particularly with respect to increasing the predictability of the regulatory framework and/or eliminating requirements that aren’t helpful?
Flexibility as a Double-Edged Sword
Kevin Crowley, director of the Nuclear and Radiation Studies Board at the National Academies, suggested that diversity and flexibility within disposal decision making is a double-edged sword. There is not much trouble handling diversity and flexibility from a technical standpoint. Where decision makers tend to fail is when they try to explain the diverse and flexible process to the people they serve. Dr. Crowley noted the importance of clearly communicating with the people who are served about the decision process: say what you are going to do, and do what you say you are going to do. Clear communication may be difficult when a system is too flexible and diverse.
Dr. Ouzounian argued that flexibility is crucially important, but it cannot be “free” flexibility. The flexibility needs to exist within a regulatory framework with clear rules, and one must be able to demonstrate that alternatives are safe and effective.
Mr. Applegate asked what a diverse and flexible framework might look like for LLW management. Mr. Garamszeghy responded that there are probably a couple approaches for establishing such a framework. One might use a performance standard, which requires a demonstration of how waste containment will be achieved. As long as the site is operated within an approved performance standard, there would be flexibility to make disposal decisions that meet that standard. This would be more flexible than a system that is based on compounding and conflicting regulations on allowable disposal options by waste type. Mr. Garamszeghy acknowledged that detailed regulations provide additional guidance to the user, but they also make it difficult to find innovative solutions when exceptions are presented.
Paul Black, chief executive officer of Neptune and Company, Inc., noted that although flexibility is critically important, cost-benefit analysis should also be considered in regulatory decisions and discussions. The current U.S. regulatory framework limits flexibility in strange ways because of competing regulatory structures. In order for the structure to change for the better, Dr. Black argued, one should strive for regulations that are simple and guidance that is process-oriented (rather than prescriptive) and based on cost-benefit considerations. The U.S. Office of Management and Budget (OMB) has the responsibility to evaluate new policies and rulemakings. As part of that evaluation, a cost-benefit analysis must be performed. OMB
has developed guidance on using cost-benefit analysis. 23 Dr. Black suggested that both DOE and the USNRC should consider this guidance.
Mr. Applegate offered ALARA 24 as an example of a cost-benefit construct. Dr. Black strongly agreed and suggested that sustainability is another example. Sustainability balances three pillars: costs/economics, sociopolitical factors, and environmental factors. Dr. Black suggested that a framework for regulatory decision-making should combine the sustainability context ( National Research Council, 2011b ) with OMB’s approach and guidance. Dr. Ouzounian noted that before cost-benefit can be assessed, safety must first be robustly demonstrated with a defense-in-depth approach.
Jennifer Heimberg, rapporteur and National Academies staff, asked Mr. Lovato whether he found it beneficial to have flexibility with the way DOE regulates over the USNRC’s approach. She asked for any specific examples that showed how DOE’s flexibility was utilized. Mr. Lovato noted that the NNSS does not have advance information about the variety of waste streams that will require disposal, so the DOE Orders are a good management structure for evaluating different types of waste streams. As an example, he cited radioisotope thermoelectric generators (strontium-90 sources originally from the Air Force) that required disposal. This waste had to be evaluated slightly differently from other waste streams; the flexibility in the DOE Orders allowed for that. However, he noted that it is always helpful to have a framework (e.g., the USNRC waste classification system) that can be used to explain waste management decisions to members of the public. Mr. Lovato was not advocating that a USNRC framework be used for DOE waste, but he cited it as the type of framework that is helpful for discussions with the public.
Elevating the Importance of Site Characteristics
Mr. Garamszeghy previously suggested that performance assessments be used as a framework for allowing flexibility in decisions while also providing boundaries. Mr. Applegate took this idea a step further by suggesting the following: One of the criticisms of the current U.S. regulatory framework is that it focuses on waste sources. What if the framework
23 “Circular A-4: Regulatory Impact Analysis: A Primer,” accessed March 27, 2017, https://obamawhitehouse.archives.gov/sites/default/files/omb/inforeg/regpol/circular-a-4regulatoryimpact-analysis-a-primer.pdf . Circular A-4 is referenced in the Trump administration’s interim guidance: https://www.whitehouse.gov/the-press-office/2017/02/02/interim-guidanceimplementing-section-2-executive-order-january-30-2017 .
24 ALARA is “as low as reasonably achievable” and refers to the practice of reducing exposure to ionizing radiation through every reasonable effort. “USNRC: ALARA,” accessed February 25, 2017, https://www.nrc.gov/reading-rm/basic-ref/glossary/alara.html .
instead focused on disposal facilities? In other words, disposal decisions would be based on whether the waste could be safely disposed of in a facility as demonstrated by a performance assessment, irrespective of the waste source. For example, for waste potentially being sent to WCS, one would ask, “What does it take to make it safe there?”
Mr. Shrum supported this idea and restated it in another form: “Consider the waste. It can go here. It can’t go there.” He noted that performance assessments have been done at all of the U.S. disposal facilities and is required under 10 CFR Part 61. But Mr. Shrum noted a potential communication problem with this approach: those whom we serve do not necessarily understand the details of a performance assessment, so they will not necessarily trust the output of the analysis. He said that the members of the public often do not understand that performance assessments are used to guide—not make—decisions. He supported Mr. Applegate’s approach, but he noted that effective ways would need to be developed to educate the public for this approach to be successful.
He also noted that scientific understanding of radioactive wastes and disposal facilities have grown significantly since the 1950s, when commercial radioactive wastes were first disposed of. Mr. Shrum argued that this new understanding must be used to inform current disposal decisions. The nuclear industry as a whole has not been very good at describing the technical rationale for disposal decisions to the public, and, Mr. Shrum believes, that will have to change as part of a new framework.
Dr. Crowley noted that the workshop was intended to focus on exceptions. There are many exceptions to the existing regulations and rules, and there are questions about the best way to handle exceptions in the future. One option is to change the rules to include the exceptions. But this is unlikely in the short term. Another option is to establish procedures to handle the exceptions, for example by establishing “mini rules” that may not be incorporated into the regulations. Those mini-rules could be implemented at disposal facilities using their WAC, which of course are based on performance assessments.
However, it is difficult to anticipate the full variety of wastes that might come to a facility during its design or construction stages. On the other hand, one could probably think about unanticipated wastes during the design and construction stages and determine how they might be handled. Facility-specific performance assessments are a reasonable way to proceed.
Mr. Applegate commented that Dr. Crowley appeared to have endorsed his idea of focusing on disposal facilities instead of the waste source. A disposal facility could develop WAC to which waste streams are matched. Dr. Crowley agreed that this approach could work as long as the analysis was done within the framework of the current regulations. A near-surface disposal facility is only going to take certain types of waste; the framework
suggested by Mr. Applegate should not be used to try to dispose of highly radioactive waste in near-surface facilities.
Dr. Black disagreed with the approach suggested by Mr. Applegate, primarily because he is not content with current regulations for radioactive waste disposal. They are overly conservative, so WACs developed using the existing regulations will also be overly constraining. For example, the inadvertent intrusion scenario in the regulations makes no sense for arid disposal sites according to Dr. Black.
Several years ago, Dr. Black developed a performance assessment for the Nevada Test Site (now NNSS), which allowed a user to enter the characteristics of a waste stream and get an answer within hours on whether it could be disposed of at the site ( DOE, 2006 and Crowe et al., 2005 ). Dr. Black argued that this is a better approach than WACs for evaluating whether a waste stream can be disposed of in a particular facility.
Taking Advantage of Knowledge Gained
Mr. Shrum previously introduced the idea of taking advantage of knowledge gained over decades of disposal operations, and Dr. Ouzounian also mentioned this idea in his case study. Scott Kirk, director of regulatory affairs at BWXT, raised this issue for further discussion, noting that the nuclear waste disposal industry has matured over the past 40 years. Modern state-of-the-art disposal facilities such as the WCS facility in Texas are remarkably different in siting and design than older disposal facilities such as Barnwell, which was state of the art in 1969. The modern sites are in arid environments, far removed from water tables, and designed with insights from 40 years of operating experience. These modern sites might be suitable for disposal of challenging LLW waste streams that could not be disposed of in older facilities. It would be useful to assess the suitability of current regulatory requirements against these modern facilities.
Charles Maguire, drector of the Radioactive Materials Division within the Texas Commission on Environmental Quality, highlighted the current state of regulations through an analogy. Most of the huge gothic cathedrals in Europe took approximately four generations to build. The last generation to work on the cathedral had little understanding of the reasons for the size, shape, or composition of the cornerstone. Yet the cathedral was built on it, and the generations of workers that followed improved their skills as cathedral construction progressed. Mr. Maguire noted that we are about to pass our nuclear knowledge on to a fourth generation of workers. But we are telling these workers to use the same tools and techniques as previous generations. We are not “getting better.”
Mr. Maguire asserted that we have to get better and to apply what we learn. We now take without question what the generation before said was
essential, and we do not apply what has been learned about mitigating risk. He concluded that we need to make sure that as we build up the structure it becomes more beautiful or practical and that we are on a path to do better. Otherwise, we may end up with a regulatory framework that no one can afford to use.
From the Outside Looking In: Public Perception
Ms. Edwards suggested that terminology is important in communicating with the public, and that the LLW classification system makes clear communications difficult. Previously, one could refer to Class A LLW as a hazard that lasted about 100 years, Class C waste as a hazard that lasted 500 years, and high-level waste as a hazard that lasted tens of thousands of years. This hazard differentiation is important because the public can become confused between high-level and low-level waste. But the 1,000-year compliance period for certain types of LLW in the proposed 10 CFR Part 61 regulation blurs the previous hazard distinctions.
Mr. Camper noted that USNRC staff were trying to address the disposal of large amounts of depleted uranium and used this opportunity to add a requirement that was not previously embodied in the regulation (but should have been). The existing 10 CFR Part 61 does not specify a period of compliance but the proposed 10 CFR Part 61 rulemaking specifies a two-tiered approach to a period of compliance, i.e., Tier 1 at 1,000 years and Tier 2 up to 10,000 years.
Mr. Garamszeghy noted that the public perceives “nuclear” and “waste” as highly dangerous in part because of the complicated and prescriptive regulations that govern them. The thought is, “It must be dangerous because there are all these regulations to protect us.”
Mr. Applegate asked Mr. Garamszeghy to expand on his presentation about compensating the communities in which the Port Hope and Port Granby LLW facilities were sited. Was there a “general sense of fairness” argument? Or was it seen as compensating for risk? Or simply paying for the privilege? Mr. Garamszeghy explained that the intent of the PVP program was never to, for lack of a better word, “buy” public support. Rather, it was recognized that building and operating the LLW facility would strain the local communities in terms of a number of new people coming in and wear and tear on public facilities, for example. The PVP program ensured that the local towns, communities, and people were no worse off after the facility was in place than they would be if the facility was not there.
Dr. Crowley commented on the recurring topic of public perceptions and communications. The term “educating the public” is often used. He finds this term to be denigrating because it suggests that the public is not educated and that, if it were, the public would agree with the experts’
conclusions—which is not always the case. Two-way communications are required to understand the concerns that the people who live around sites have about those sites.
Dr. Ouzounian noted that the term “stakeholders” is no longer used in France. Rather, the terms “concerned” or “interested parties” are used because this involves all parties, including waste producers and academics.
He also noted that the French Parliament passed a law in July 2016 as the result of a public debate on social benefits and responsibilities. The current generation benefits from the electricity generated by nuclear power plants, so it should be responsible for solving the waste management problem for following generations. The law required that a master plan describing all the major milestones of the lifetime of each disposal facility be developed and periodically reviewed. Initially, the planned review period was 10 years. However, Parliament decided that reviews will occur every 5 years with the involvement of all concerned or interested parties.
Dr. Ouzounian also commented on compensation to local communities. Compensation is provided because of expected damage to the infrastructure and the environment, resulting for example from large numbers of trucks on the roads during construction, not from increased risk. Parliament had another important debate in 2006. One side was arguing that nuclear industries were “buying the public” by giving money to communities. The other side was argued by the high commissioner for nuclear power in France. He pointed out that one community will accept the waste that belongs to all French people benefitting from electricity. This one community shows their solidarity with the country. He argued that, therefore, it was the responsibility of the rest of France to also show solidarity by supporting the community in developing its territory and its activities. This latter argument was accepted by the Parliament and ended comments about “buying the people.”
Dr. Black also commented on communication and public perception. He recalled that Mr. Shrum said that issues with LLW are more political than technical. The politics really come down to stakeholders, which means everyone associated with the disposal facility or the potential facility and the affected communities. The different outcomes for the Yucca Mountain and WIPP facilities provide a good example. In both cases, decisions on facility siting and construction were influenced by stakeholders and the political environment rather than the technical analyses. Dr. Black believes it is important to understand and “get on top of” the stakeholder issues before addressing regulatory change.
Mr. Camper spoke about the evolution of stakeholder engagement on USNRC decisions. Earlier in his career at the USNRC, staff would create new regulations and guidance documents without public input. But that changed over time for a number of reasons, not the least of which were
regulatory failures. Stakeholders and interested parties demanded that decisions not be based entirely on the USNRC’s scientific analyses. These demands have changed the way new regulations are developed and released.
“Regulatory Morass” Redux
Dr. Black commented that the “regulatory morass” that he referred to previously includes TRU waste. Defense TRU waste must be disposed of at WIPP, a deep geologic repository, but commercial waste containing less than 100 nCi/g of TRU nuclides can be disposed of in a near-surface disposal facility meeting the requirements of 10 CFR Part 61. Also, there are multiple regulations from DOE, USNRC, EPA, and the states for disposal facilities, some of which overlap or are in conflict.
This page intentionally left blank.
The Department of Energy's Office of Environmental Management (DOE) is responsible for the safe cleanup of sites used for nuclear weapons development and government-sponsored nuclear energy research. Low-level radioactive waste (LLW) is the most volumetrically significant waste stream generated by the DOE cleanup program. LLW is also generated through commercial activities such as nuclear power plant operations and medical treatments.
The laws and regulations related to the disposal of LLW in the United States have evolved over time and across agencies and states, resulting in a complex regulatory structure. DOE asked the National Academies of Sciences, Engineering, and Medicine to organize a workshop to discuss approaches for the management and disposition of LLW. Participants explored the key physical, chemical, and radiological characteristics of low-level waste that govern its safe and secure management and disposal in aggregate and in individual waste streams, and how key characteristics of low level waste are incorporated into standards, orders, and regulations that govern the management and disposal of LLW in the United States and in other major waste-producing countries. This publication summarizes the presentations and discussions from the workshop.
READ FREE ONLINE
Welcome to OpenBook!
You're looking at OpenBook, NAP.edu's online reading room since 1999. Based on feedback from you, our users, we've made some improvements that make it easier than ever to read thousands of publications on our website.
Do you want to take a quick tour of the OpenBook's features?
Show this book's table of contents , where you can jump to any chapter by name.
...or use these buttons to go back to the previous chapter or skip to the next one.
Jump up to the previous page or down to the next one. Also, you can type in a page number and press Enter to go directly to that page in the book.
Switch between the Original Pages , where you can read the report as it appeared in print, and Text Pages for the web version, where you can highlight and search the text.
To search the entire text of this book, type in your search term here and press Enter .
Share a link to this book page on your preferred social network or via email.
View our suggested citation for this chapter.
Ready to take your reading offline? Click here to buy this book in print or download it as a free PDF, if available.
Get Email Updates
Do you enjoy reading reports from the Academies online for free ? Sign up for email notifications and we'll let you know about new publications in your areas of interest when they're released.

Press centre
Nuclear technology and applications.
- Climate change
- Environment
- Food and agriculture
- Nuclear science
Nuclear safety and security
- Human and organizational factors
- Governmental, legal and regulatory framework
- Nuclear installation safety
- Radiation protection
- Security of nuclear and other radioactive material
- Radioactive waste and spent fuel management
- Emergency preparedness and response
Safeguards and verification
- Basics of IAEA Safeguards
- Safeguards implementation
- Safeguards legal framework
- Assistance for States
- Member States Support Programmes
Technical Cooperation Programme
- How it works
- How to participate
Coordinated research activities
- Legislative assistance
Key programmes
- Atoms4NetZero
- International Project on Innovative Nuclear Reactors and Fuel Cycles (INPRO)
- Together for More Women in Nuclear
- NUTEC Plastics
- Peaceful Uses Initiative
- Rays of Hope
- The SMR Platform and Nuclear Harmonization and Standardization Initiative (NHSI)
- Zoonotic Disease Integrated Action (ZODIAC)
Review missions and advisory services
- Catalogue of review missions and advisory services
- Peer review and advisory services calendar
Laboratory services
- Analytical reference materials
- Dosimetry calibration
- Dosimetry auditing
- Inter-laboratory comparisons
- Global Nuclear Safety and Security Network (GNSSN)
Education and training
- Training courses
- Online learning
Scientific and technical publications
- Full catalogue
- Safety Standards
- Nuclear Security Series
- Nuclear Energy Series
- Human Health Series
- Conference Proceedings
- Newsletters
- Nuclear Fusion Journal
General interest material
- IAEA Bulletin
- Nuclear Explained
- Photos (Flickr)
- Photo essays
- Briefs and factsheets
- IAEA Virtual Tours
Official documents
- Information circulars
NUCLEUS information resources
- International Nuclear Information System (INIS)
- Power Reactor Information System (PRIS)
- Advanced Reactors Information System (ARIS)
- Integrated Nuclear Fuel Cycle Information System (iNFCIS)
- Spent Fuel and Radioactive Waste Information System (SRIS)
- Nuclear Data Services (NDS)
- Research Reactor Database (RRDB)
Other resources
- Library – Nuclear Information Services
- Impact stories
- Press releases
- Media advisories
- Director General statements
- Photo library
- Press contacts
- Press enquiries
- General Conference
- Board of Governors
- Scientific and technical events
- Scientific Forum
- Medium-Term Strategy
- Partnerships
- Gender at the IAEA
- Sustainable Development Goals (SDGs)
- Multilingual content
- List of Member States
Management team
- Director General
- Deputy Directors General
Organizational structure
- Offices Reporting to the Director General
- Technical Cooperation
- Nuclear Energy
- Nuclear Safety and Security
- Nuclear Sciences and Applications
- Working at the IAEA
- Types of Employment
Procurement
- Procurement overview
Search form
You are here.
- Publications
Addressing Challenges in Managing Radioactive Waste from Past Activities
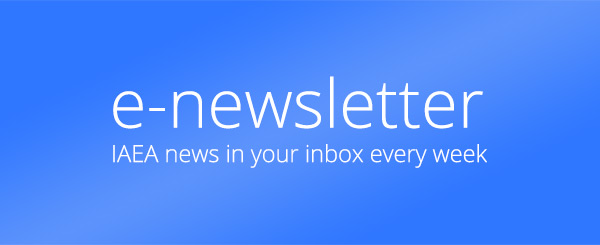
If you would like to learn more about the IAEA’s work, sign up for our weekly updates containing our most important news, multimedia and more.
- Arabic (monthly)
- Chinese (monthly)
- English (weekly)
- French (monthly)
- Russian (monthly)
- Spanish (monthly)
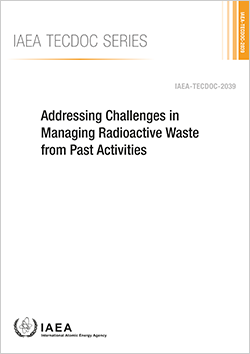
IAEA-TECDOC -2039
English IAEA-TECDOC-2039 ¦ 978-92-0-102724-5
Download PDF (2.8 MB)


Supplementary files
Supplementary data.
ID | Title | Excel | CSV |
---|---|---|---|
Cite this content as:
INTERNATIONAL ATOMIC ENERGY AGENCY, Addressing Challenges in Managing Radioactive Waste from Past Activities, IAEA-TECDOC-2039, IAEA, Vienna (2024)
Download to: EndNote BibTeX *use BibTeX for Zotero
Description
The publication aims to support the safe and effective management of radioactive waste from past activities by presenting focused information on the specific challenges associated with such waste and providing guidance on how to develop and implement strategies to address those challenges. The main objective is to describe typical characteristics of legacy wastes and identify the major challenges to their safe management. In support, information gained through analysis of varied experiences, case studies, good practices and lessons learned are given, aiming to provide guidance on strategies for Member States to overcome these challenges, successfully manage their existing legacy waste inventories, and minimize the risk of their creation in the future. The information provided will be of interest to the waste management community, particularly to those responsible for the safe and effective management of radioactive waste from past activities in their respective countries.
More Information on reusing IAEA copyright material.
Related publications

Modelling Approaches for Management and Remediation at Sites Affected by Past Activities
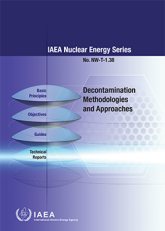
Decontamination Methodologies and Approaches

Determination of Environmental Remediation End States

Ten Years of Remediation Efforts in Japan

Assessment of Radioactive Contamination and Effectiveness of Remedial Measures in Urban Environments

Remediation Strategy and Process for Areas Affected by Past Activities or Events
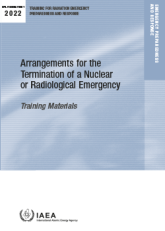
Arrangements for the Termination of a Nuclear or Radiological Emergency

Case Study on Assessment of Radiological Environmental Impact from Potential Exposure

Strategies and Practices in the Remediation of Radioactive Contamination in Agriculture

Developing Cost Estimates for Environmental Remediation Projects

Advancing the Global Implementation of Decommissioning and Environmental Remediation Programmes

Advancing Implementation of Decommissioning and Environmental Remediation Programmes

Policy and Strategies for Environmental Remediation
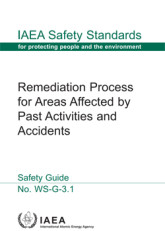
Remediation Process for Areas Affected by Past Activities and Accidents
Ordering Locally
Please use the following links for an up-to-date list of IAEA distributors:
Distributors of IAEA Publications
How to Access IAEA e-books
Orders and requests for information may also be addressed to:
Publishing Section International Atomic Energy Agency Vienna International Centre PO Box 100, A-1400 Vienna, Austria
Tel.: +43 1 2600 22529, +43 1 2600 22530 Email: [email protected]
Download order form
More on the IAEA
- Privacy Policy
- Logo Usage Guidelines
Scientific resources
- Information Circulars
- Standards and guides
- Safeguards and Additional Protocol
Stay in touch
Nuclear Decommissioning Case Studies: Characterization, Waste Management, Reuse and Recycle
A summary of the sustainability of nuclear decommissioning.
- 1st Edition, Volume 6 - July 20, 2023
- Editor: Michele Laraia
- Language: English
- Paperback ISBN: 9780323918497 9 7 8 - 0 - 3 2 3 - 9 1 8 4 9 - 7
- eBook ISBN: 9780323919470 9 7 8 - 0 - 3 2 3 - 9 1 9 4 7 - 0
Nuclear Decommissioning Case Studies: Characterization, Waste Management, Reuse and Recycle, Author’s Statement on the Sustainability of Nuclear Decommissioning, Volume Six p… Read more
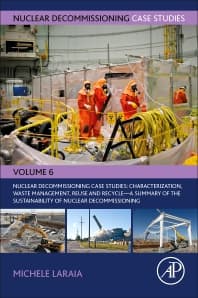
Purchase options
Limited Offer
Save 50% on book bundles
Immediately download your ebook while waiting for your print delivery. no promo code is needed..
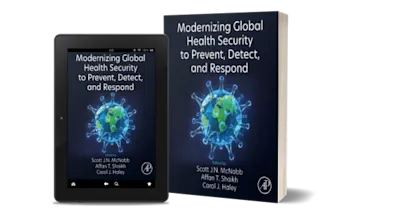
Institutional subscription on ScienceDirect
Nuclear Decommissioning Case Studies: Characterization, Waste Management, Reuse and Recycle, Author’s Statement on the Sustainability of Nuclear Decommissioning, Volume Six presents a selection of global case studies that focus on a range of technologies for the decontamination, dismantling, spent fuel treatment and recycling of nuclear decommissioning. The book presents best practices by analyzing errors and inadequacies, leading the reader to sound decision-making. The events covered in this publication range from national and local legislation, to regulatory positions, statements or recommendations, licensing steps and transition phases.
Decommissioning experts, including regulators, operators, waste managers, researchers and academics will find this book to be suitable supplementary material to Michele Laraia’s reference works on the theory and applications of nuclear decommissioning. Alongside the other case studies books in this series, readers will obtain an understanding of various key case studies-what happened and what we can learn from them, to help supplement, solidify and strengthen their understanding of the topic.
- Presents a selection of global case studies which focus on the characterization, waste management, and reuse/recycling options in nuclear decommissioning
- Highlights important sustainability and socioeconomic factors associated with the abovementioned decommissioning aspects
- Based on experience and lessons learned, guides the reader to ensure the most appropriate and efficient technologies and strategies are utilized in order to pursue adequate sustainability
- Addresses all aspects of decommissioning with a bottom-up, inductive approach, aimed to elicit general conclusions about sustainability from individual case studies
Nuclear engineers; nuclear researchers; nuclear students; decommissioning specialists; governments and decision makers; nuclear operators and waste managers
1. Introduction 2. The concept of environmental sustainability as applicable to nuclear decommissioning (as applicable to this book) 3. The structure of this book: Radiological and physical characterization, decontamination and dismantling, waste management 4. Errors, mishaps, inadequacies due to improper technologies (conversely, best practices) 5. Characterization Case Studies 6. Decontamination and Dismantling Case Studies 7. Waste Management Case Studies 8. Conclusions
- No. of pages : 474
- Language : English
- Edition : 1
- Published : July 20, 2023
- Imprint : Academic Press
- Paperback ISBN : 9780323918497
- eBook ISBN : 9780323919470
Michele Laraia
Warning: The NCBI web site requires JavaScript to function. more...

An official website of the United States government
The .gov means it's official. Federal government websites often end in .gov or .mil. Before sharing sensitive information, make sure you're on a federal government site.
The site is secure. The https:// ensures that you are connecting to the official website and that any information you provide is encrypted and transmitted securely.
- Publications
- Account settings
- Browse Titles
NCBI Bookshelf. A service of the National Library of Medicine, National Institutes of Health.
National Academies of Sciences, Engineering, and Medicine; Division on Earth and Life Studies; Nuclear and Radiation Studies Board; Planning Committee on Low-Level Radioactive Waste Management and Disposition: A Workshop. Low-Level Radioactive Waste Management and Disposition: Proceedings of a Workshop. Washington (DC): National Academies Press (US); 2017 Apr 13.

Low-Level Radioactive Waste Management and Disposition: Proceedings of a Workshop.
- Hardcopy Version at National Academies Press
3 Successful Disposition Case Studies
Rebecca Robbins, planning committee member and predisposal unit head at the International Atomic Energy Agency (IAEA), moderated this session, which used case studies to highlight examples of successful low-level waste (LLW) management and disposal within current regulatory frameworks. The case studies presented situations in which previously challenging LLW streams 1 were successfully managed and disposed of. The first two presentations in this session provided case studies from the United States; the next two presentations focused on case studies from outside the United States. A discussion was held after all of these case studies had been presented.
The comments from the moderators, the panelists, and other workshop participants are their own. They do not necessarily represent official views of their employers, governments, or other organizations that may be mentioned in the presentations or discussions.
Dr. Robbins began the session by requesting the workshop participants, as they listened to each case study, to identify the “key characteristics” that contributed to its success. Key characteristics include the practices, activities, attitudes, and actions with respect to the case studies and associated regulatory frameworks.
Melanie Pearson Hurley, headquarters liaison in the Office of Field Operations within the Department of Energy (DOE), presented a DOE case study. Greg Lovato, deputy administrator at the Nevada Division of Environmental Protection (NDEP), provided examples of key characteristics for successful disposition from the perspective of a state regulator. For international case studies, Miklos (Mike) Garamszeghy, design authority and manager of technology assessment and planning for the Canadian Nuclear Waste Management Organization, provided two examples from Canada and Gérald Ouzounian, international director for the National Radioactive Waste Management Agency (ANDRA), provided a case study from France.
3.1. UNITED STATES CASE STUDIES
Case study 1: separations process research unit tank waste sludge.
Mrs. Hurley presented the Separations Process Research Unit (SPRU) project as DOE's case study. In the early 1950s, research on plutonium and uranium separation techniques such as PUREX and REDOX 2 was performed at SPRU within the Knolls Atomic Power Laboratory (KAPL). 3 KAPL, now an active naval nuclear laboratory, is located near Schenectady, New York, adjacent to the Mohawk River. The inactive SPRU facilities occupy about 5 acres of land immediately adjacent to KAPL.
The research at SPRU was performed on a laboratory scale and supported larger operations at both the Hanford Site in Washington and the Savannah River Site in South Carolina. Radioactive liquid and sludge wastes resulting from the SPRU research were stored in seven tanks located on site. The SPRU project timeline was established by the demolition dates for the buildings in which the research was performed and the wastes were stored. There was a strict requirement that the sludge waste be removed and disposed of by spring 2014.
Figure 3-1 provides a cross-section and plan view of two facilities at SPRU. The top drawing is a cross-section of the G2 building, which housed the laboratories, hot cells, and separations processing and testing equipment, and the H2 building, which was used for liquid and solid waste processing. The G2 and H2 buildings are connected by an underground tunnel. The lower drawing in Figure 3-1 shows the plan view of buildings G2 and H2. The tank farm in the lower-right corner of the figure is the focus of this presentation.
Schematic of SPRU facility showing cross-sections (top drawing) and plan views (bottom drawing) of Buildings G2 and H2. SOURCE: Courtesy Jeff Selvey, AECOM.
The radioactive waste from chemical processing was stored in the H2 tank farm (seven underground concrete-enclosed stainless steel tanks). This waste included about 200 cubic feet (5.7 cubic meters) of sludge consisting of fine particulates and liquids containing fission products, mostly cesium and strontium, and long-lived transuranic (TRU) radionuclides, primarily plutonium-239. The sludge contained 36 curies of total radionuclides, including 2.5 to 6.5 curies of TRU radionuclides. The concentration of the long-lived TRU radionuclides in the final waste packages ranged from 11.5 to 65.5 nanocuries per gram (nCi/g).
The total mercury content of the sludge was more than 1 percent, and it contained high levels of lead, chromium, and cadmium. This led to an initial determination that the sludge may be a Resource Conservation and Recovery Act (RCRA) characteristic hazardous waste 4 for metals. This waste classification would have complicated the management of the sludge because the hazardous component would be regulated by the Environmental Protection Agency (EPA) in addition to DOE's regulation of the radioactive component. However, two toxicity characteristic leaching procedures (TCLP) 5 confirmed that the hazardous component of the waste was only at 0-3 percent of regulatory levels, due to the low solubility of the metals in the sludge. Consequently, the sludge was determined to not contain hazardous waste and could more simply be managed under DOE orders.
DOE Order 435.1, Radioactive Waste Management , was used to guide decisions on disposing of the sludge from SPRU. The Order allows for the disposition of LLW in federal or commercial facilities. An exemption request must be approved by DOE headquarters for waste to be disposed of in a commercial disposal facility. Approval will be given if commercial disposal demonstrates compliance with regulations and waste acceptance criteria (WAC), is cost-effective, and is determined to be “in the best interests of the United States government.”
There were two disposal options for the SPRU sludge: the Nevada Nuclear Security Site (NNSS), a DOE disposal site in Nevada, and Waste Control Specialists (WCS), a commercial disposal site in Texas. Both disposal options were explored, and WCS was selected, in part due to the compressed schedule 6 for completing cleanup of the SPRU tanks (spring 2014).
DOE worked closely with Texas regulators and WCS on establishing the waste profile 7 through the standard process described in the WCS Waste Acceptance Plan. 8 Texas regulators accepted DOE's policy that waste is not formally classified until all processing is completed and a stabilized waste form is produced. Mrs. Hurley identified this close collaboration as a “key characteristic” for successful disposition of the sludge.
The plan was to have the waste stabilized using a mixture of cement, fly ash, and slag that was then solidified in the final waste package for transportation and final disposal. The sludge solidification system at SPRU was designed and cold tested off site by the vendor and then installed in the H2 tank vault area. Cold-test operations were conducted on site prior to hot operations to ensure the system would perform as designed.
Figure 3-2 is a schematic of the H2 tank vault area and processing systems. Mrs. Hurley noted that there was an airborne release of radioactive material at SPRU in 2010. As a consequence of this event, the EPA required DOE to construct a tent enclosure over the H2 facility with portable ventilation units (contained in the outer tent, Area H2 Tent, shown in Figure 3-2 ). Underneath this larger tent is another tent (Existing Big Top Tent in Figure 3-2 ) that originally served as a weather enclosure over the tank farm. This weather-enclosure tent was retained when the larger enclosure was constructed to add another level of protection.
Schematic of the H2 tank vault area including SPRU processing contamination enclosures consisting of the outer enclosure (Area H2 Tent), the existing Big Top Tent, and two smaller tents for the sludge waste retrieval and processing (the Tank Containment (more...)
Within the Big Top Tent are two additional tents, the Tank Containment Retrieval and Solidification Containment Tents (see Figure 3-2 ). The sludge retrieval, mixing, processing, and characterization operations were carried out in these tents. Batches of sludge were retrieved from the 509E Tank, 9 mixed to suspend the solids in the waste, transferred to the final waste package, and then combined with cement, fly ash, and slag. The mixture was periodically checked by a penetration test to determine when it was solidified. If there was any remaining free water, additional cement mix was added.
The waste package was moved into a shielded temporary storage area set up in the G2 building ( Figure 3-1 ). 10 The cement mixture curing times were long because the storage area was unheated. Once fully cured, the waste packages were shipped to WCS for disposal.
Sludge processing began on September 9, 2013, and the final shipments to WCS were completed on February 27, 2014. 11 Nearly 10,000 gallons of sludge were processed and solidified in 28 liners. The liners were shipped to WCS via trucks. (There were two liners per truckload and a total of 14 truck shipments.) This campaign removed the majority of the radionuclides from the SPRU site and allowed DOE's deactivation activities to continue in the H2 basement as scheduled.
While this case study highlights many successes, there were obstacles to overcome, including the following:
- Working within a decades-old facility with limited physical and onsite storage. There was no lay-down area where more than one liner could cure at the same time, and the temporary storage area in the G2 building allowed for 3 to 4 liners at a maximum.
- Retrieving sludge from the 509E Tank, including cleaning out solids near the bottom of the tank.
- Working with a waste stream (sludge) that is difficult to characterize and process. A continuous mixing system was used to keep solids suspended in the waste so that the final waste form was homogenous.
- Performing the sludge processing work immediately adjacent (less than 25 feet or 7.6 meters) to a currently operating research and development laboratory and during deconstruction of the G2 building.
- Performing this work in a tent-type containment structure ( Figure 3-2 ). Portable ventilation units and the HEPA 12 filters were used to ensure that safe working conditions were maintained.
- Addressing waste classification uncertainties. DOE performed historical research and additional evaluations to show that the sludge waste was not high-level waste and could be managed as LLW.
Several key management practices contributed to the success of this project:
- A dedicated and technically competent workforce that understood the mission objective and the importance of safety, including an excellent DOE federal project director.
- Frequent communications among the DOE participants, DOE staff from headquarters, NNSS, DOE's consolidated business center in Cincinnati, and KAPL, the adjacent research and development laboratory. Support from a “Senior Integrated Project Team” was also key to the success of the project.
- Cold testing of the treatment system at the vendor site and on site prior to operation enabled the right combination of nozzles, sluicing, and camera angles to confirm that the solids were removed from the 509E Tank.
- Early and frequent communication and engagement with the waste disposal experts from WCS.
- Coordination with the expertise throughout the DOE complex on packaging and transportation.
A participant asked Mrs. Hurley how DOE verified that solidification was adequate during cold testing. She responded that the cold testing was primarily to confirm the pump's ability to mix the solids and liquids and to confirm homogeneous mixing. Solidification was not tested or verified during cold testing; rather, a cement and fly ash “recipe” that was used successfully at other sites was used to solidify the SPRU sludge.
Case Study 2: Low-Level Radioactive Waste Streams Reviewed for Disposal at the NNSS: Key Characteristics, Variation, and Management
Mr. Lovato's presentation included an overview of the waste disposal sites at the NNSS, the waste profile review process, key waste stream characteristics and their variation, and key management steps taken to address some of those different characteristics.
Mr. Lovato explained that NDEP was participating in the workshop because of a memorandum of understanding between the governor of Nevada and the secretary of DOE. One of the goals of the agreement is to hold a workshop to bring more transparency and predictability to DOE's waste disposal decisions. Mr. Lovato expressed thanks that the workshop was taking place. He noted the desire by Nevada citizens for context and predictability in DOE disposal decisions and asked the workshop participants for help in developing a LLW classification system that would foster greater confidence in future disposal decisions; he also admitted that these requests were tall orders.
Mr. Lovato suggested one way to think about Nevada's participation in this workshop is illustrated by a famous line from the movie Jerry Maguire , in which the sports agent, played by Tom Cruise, is trying to negotiate a contract for a professional athlete, played by Cuba Gooding, Jr. The sports agent repeatedly asks the athlete to “Help me, help you.” The goal of the memorandum of understanding between Nevada and DOE is to “Help us, help you.”
The NNSS is located about 65 miles northwest of Las Vegas. The Area 5 disposal facility is a secure, 740-acre site located in the southeast corner of the NNSS (see Figure 3-3 ). The disposal facility is used to dispose of mixed LLW 13 under a RCRA permit with the state of Nevada. The waste is disposed at depths of up to 24 feet (7.3 meters).
Maps of the NNSS (left image) and the location of Area 5 (middle image), and Area 5's active (yellow) and closed (gray) cells (right image). SOURCE: Modified from DOE Office of Environmental Management.
Area 5 receives less than 5 inches (13 centimeters) of annual rainfall, and depth to groundwater is 770 feet (235 meters). Infiltration of precipitation below the plant root zone ceased between 10,000 and 15,000 years ago. Consequently, migration of the waste to groundwater is less of a risk than surface erosion from thunderstorms.
NNSS accepts approximately 1.0-1.5 million cubic feet (28,000-43,000 cubic meters) of LLW, mixed LLW, and classified waste 14 per year from more than 25 different DOE facilities. This amounts to between 5 and 10 percent of the volume of wastes disposed of across the DOE complex, including DOE wastes disposed of at commercial disposal sites ( Marcinowski, 2016 ).
NDEP is a member of the Waste Profile Review Team. The team includes DOE, contractors, and three members of NDEP and meets weekly to review waste profiles against the NNSS WAC. If a waste stream does not meet the WAC, it will not necessarily be rejected for disposal at the NNSS. The performance assessment for the facility can be reanalyzed to determine whether the waste stream under consideration would meet the facility's performance objectives.
LLW can have a broad spectrum of characteristics. Table 3-1 provides a list of key characteristics of the LLW and mixed-waste streams considered for disposal at the NNSS. (This list was developed by Mr. Lovato based on his experiences at the NNSS.) The table shows that these waste streams have a wide range of half-lives, activities (expressed as a ratio to WAC thresholds), and plutonium equivalent grams.
Variation of Key Characteristics in NNSS LLW Profiles.
Using a “plutonium equivalent grams” (PE-g) is a way to normalize the activity of different isotopes in a single package to a single standard (the activity of plutonium-239). This normalization allows for the easy determination of whether a package meets the WAC for the NNSS. (The WAC specifies a PE-g limit for each package.) The WAC for the Waste Isolation Pilot Plant (WIPP) also contains a plutonium equivalency criterion. The list of radionuclides in the WAC for the NNSS is far longer than that for WIPP, suggesting that the NNSS deals with a more diverse range of waste streams. In fact, waste characteristics at the NNSS can have a 6-17 order-of-magnitude range in values (see Table 3-1 ).
Waste management decisions are usually handled on a case-by-case basis to ensure that waste streams are appropriate for disposal at the NNSS and that stakeholder concerns are addressed. Some of the management steps used at the NNSS include decisions to adjust burial depth or transportation routing, conducting exercises in outreach and notification, and ensuring conditions on any waste profile approvals are met.
Case-by-case decisions can seem ad hoc, subjective, and reactive without a reference system to compare the decisions to—especially when viewed from the outside. Nevada is interested in facilitating alternatives to disposal at the NNSS, for example by the preventing waste streams from being created and finding alternative disposal locations.
Mr. Lovato suggested a potential categorization scheme for LLW that could aid in final disposition decisions ( Table 3-2 ). This scheme proposes a few key physical, chemical, and radiological characteristics and hazards of LLW that should be considered for its safe and secure management and disposal. Also included are key characteristics of a disposal site (i.e., location, security, and control options such as inherent and engineered barriers of a site). A new regulatory framework would break down these characteristics based on the variety of potential LLW streams and transparently list the proposed disposal criteria.
Potential Categorization Scheme of LLW to Guide Disposition Decisions.
Mr. Lovato suggested that the regulatory framework should be scalable when considering new LLW streams: concerns about the new LLW stream from the waste generators, recipients, public, and DOE should be captured; options for addressing those concerns should be identified using characteristics similar to those in Table 3-2 ; and options for the management and disposal of a new LLW stream should be compared against each other in a transparent way. The idea is that this new framework could be created a priori without having knowledge of the LLW streams. This type of regulatory framework would be helpful in providing context on LLW disposal decisions.
Mr. Lovato encouraged the participants not to lose heart in terms of trying to develop a better LLW categorization scheme. He acknowledged that past LLW disposal decisions were likely made for expediency and were weighed against what disposal options and regulatory frameworks were available at the time. But it is incumbent upon us in the present day to improve the system, so that future stakeholders have much-needed context for the decision-making process, which may ultimately improve stakeholder confidence in LLW management and disposal decisions.
Dr. Robbins asked a clarifying question related to Nevada's desire to facilitate alternatives to the creation of waste streams. Was there a particular waste stream that does not fall within the NNSS' remit to accept? If so, can the NNSS discuss the possible acceptance of this waste stream with the waste generator?
Mr. Lovato explained that it is important to the NNSS and Nevada to not only look for alternative disposal options, but also alternative technologies for generating wastes. For example, the NNSS is seen as the disposal facility for sealed sources. But in Nevada's view, disposal of sealed sources should not default to a single location. So, Nevada is considering alternatives, such as reducing the use of sealed sources to begin with or by considering alternative disposal pathways, so that the NNSS is not relied on for disposal of all sealed sources.
3.2. INTERNATIONAL CASE STUDIES
Case studies 3-4: two low-level waste case studies from canada.
Mr. Garamszeghy's presentation was split into three parts: background on Canadian nuclear regulation and management, a case study on the Port Hope Area Initiative (PHAI), and a case study on the Deep Geological Repository for low- and intermediate-level wastes. The PHAI disposal facility is currently under construction. The Deep Geological Repository facility for low- and intermediate-level wastes is still in the regulatory approvals phase.
There are 19 operational power reactors at four sites in Canada (three sites in Ontario and one in New Brunswick). All are CANDU 15 pressurized heavy water reactors, and all are owned by the provincially owned electric utilities (Ontario Power Generation [OPG] and New Brunswick Power). Eight of the reactors in Ontario are leased to a private firm for operation, but OPG retains the responsibility for the waste produced by these reactors and for their decommissioning. There are seven other power reactors in Canada in different stages of decommissioning. There are also seven research reactors in Canada, two reactors (one operating, the other shut down) at the Canadian Nuclear Laboratories (located near Chalk River, Ontario) and the others at universities. 16 There are numerous other historic and legacy sites undergoing decommissioning or remediation.
The Canadian Nuclear Safety Commission (CNSC) is the federal nuclear regulator, equivalent to the U.S. Nuclear Regulatory Commission (USNRC) in the United States. Unlike Agreement States in the United States, the CNSC has not devolved any regulatory responsibilities to Canadian provinces. 17 The Canadian Environmental Assessment Agency (CEAA) is the federal agency responsible for the environmental assessment process. In the past there was a Joint Review Panel, which was a project-specific panel set up jointly by the CNSC and the CEAA, to review environmental assessment applications and specific license applications. This process is no longer used for nuclear projects. The proponent or the project owner/operator also has responsibilities as the eventual license holder. The proponents prepare the environmental assessment, the safety report, and the thousands of pages of support documentation.
The CNSC takes its authority from the Nuclear Safety and Control Act of 2000. It is a “quasi-judicial administration tribunal” that reports directly to Parliament. The commission members are independent and mostly part-time. All of the commission hearings are open to the public and are webcasted.
The CNSC has federal jurisdiction over both nuclear facilities and activities, much the same as the USNRC. It also provides regulatory oversight of all the licensees and disseminates objective scientific, technical, and regulatory information to the public—a fairly important role when it comes to public engagement for nuclear- and waste-related projects. The decisions of the CNSC can only be challenged through judicial review in federal court. The CNSC's decision making is transparent and science-based, at least in theory.
Risk assessments that apply to waste disposal include both a normal evolution scenario (climate change and gradual loss of engineered barriers) and disruptive scenarios (such as human intrusion). The assessment timeframe encompasses the time of maximum calculated impact (e.g., peak dose). In the case of a radioactive waste disposal facility, that time may be several million years in the future. The dose constraint for the normal evolution scenario is 0.3 milliseiverts per year (mSv/yr), equivalent to 30 millirem per year (mrem/yr). For disruptive scenarios, it is usually only a guideline of 1 mSv/yr (or 100 mrem/yr).
Canada has several types challenging LLW streams including:
significant amounts of carbon-14 from CANDU reactors,
irradiated/activated zirconium and niobium hardware from reactor refurbishments,
high-activity cobalt-60 waste, and
stored tritium (each storage canister holds about half a million curies of tritium).
- Waste from small waste generators who may have difficulty identifying disposal pathways, especially for intermediate-level waste; and
- Large volumes of historic wastes, of which characteristics and quantities not always well documented.
The PHAI will dispose of approximately 2 million cubic meters of waste, mostly soils, in engineered mound-type facilities with multicomponent caps. This disposal will take place in two locations near Port Hope and Port Granby, located east of Toronto. The Port Hope facility is expected to be in operation in 2017; the Port Granby facility is expected to be in operation in 2018. Most of the wastes to be disposed of in these facilities are located at these facilities or nearby.
The history of the sites that are hosting these facilities can be seen in Box 3-1 . The Port Hope site was used first for radium refining and later for uranium refining. These activities contaminated the site and produced large volumes of waste. A task force was established in 1988 to find a site in Canada to dispose of the Port Hope wastes. The task force was unable to reach an agreement with a community in Canada to host a site primarily because of concerns about transporting large volumes of radioactive waste.
History of Port Hope and Port Granby sites.
In 1997, Hope Township initiated a proposal to construct a long-term waste management facility near the Port Hope site. The PHAI was initiated in 2001, and environmental assessments were completed for Port Hope and Port Granby projects by 2009. Part of the agreement includes the Property Value Protection (PVP) program, which will compensate homeowners should the value of their property be reduced by the presence of the facilities.
The CNSC granted the construction license for the facility in Port Hope in 2009 and a construction license for Port Granby in 2011. The federal government made a major commitment of more than $1 Canadian billion to fund the construction of the two sites in 2012.
The Deep Geological Repository for low- and intermediate-level waste will be used to dispose of OPG-owned waste (i.e., waste from the operation and maintenance of OPG-owned facilities). The repository site is located near the Bruce Nuclear Generating Station on the eastern shore of Lake Huron in Ontario.
The community near the Bruce station volunteered to host the disposal facility. The community preferred that a single facility be used to dispose of all of OPG's waste. Accordingly, a deep geologic repository was designed for co-disposal of low- and intermediate-level wastes. A near-surface facility would not have been able to accept all of the intermediate-level wastes currently stored on the site. Also, a single deep geologic repository is less costly than building two separate disposal facilities.
The repository has a design capacity of about 200,000 cubic meters as packaged for disposal at a reference depth of 680 meters. Operation was originally expected to begin in the mid-2020s. The repository is currently in the regulatory review process (which is taking longer than the originally scheduled 2 years).
The official hosting agreement was signed in 2004 and was approved by the community in 2005 based on an independent poll of all year-round and seasonal residents. 18 It provides approximately $30 million in compensation to both the official host town (Kincardine) and other surrounding communities. The compensation is tied to project milestones until the repository construction is complete. After disposal operations begin, the compensation is akin to an annual fee.
The environmental assessment and licensing documentation was submitted to the CSNC in April 2011, but Canadian federal elections delayed the appointment of the Joint Review Panel until January 2012. The Joint Review Panel then implemented a public comment period that was originally planned to last for 90 days. However, the period was repeatedly extended and lasted for more than 1 year. There were, in total, 31 days of public hearings, which created 20,000 pages of documentation and more information requests from the Joint Review Panel and public. The Panel's report was submitted to the CSNC in May 2015; it strongly recommended the repository proceed to the licensing phase.
CEAA then held a public comment period. A decision by the Minister of Environment was expected in September 2015 but was subsequently extended to December. Another Canadian federal election in fall 2015 resulted in a change in government. The new minister asked for more work to be done. The responses to the minister's request are expected to be submitted by the end of 2016 with a final decision by the minister on the environmental assessment in early 2017. 19 If the minister approves the project it will move to the licensing phase.
This project has had several successes. Throughout the public review—with extensive local, national, and international scrutiny—the scientific evidence remained sound and passed all credible challenges. Despite a number of changes in government, local leadership, and residents, the politicians and the local community remained supportive. The project delays have allowed some opposition groups in Canada and the United States to organize and gain some support. Some members of the public became confused between two nuclear waste disposal projects planned in the same area, one for OPG's low- and intermediate-level waste and the other for spent fuel. Public outreach continues, and OPG continues to respond to public questions and concerns. The formal decision by the Minister will define the project's next step.
Case Study 5: The French Case: Low-Level Radioactive Waste Management
Dr. Ouzounian's case study provided insight into the French approach to disposing of very low-level waste and LLW. He noted that his presentation focused mostly on the LLW because it is more challenging and more interesting in terms of approach and process.
ANDRA is responsible for the long-term management of all radioactive waste produced in France. The agency is independent from waste producers and reports to ministers in charge of the environment, energy, and research. It has approximately 650 employees with an annual budget of €250 million. ANDRA's work is performed within the framework of the Planning Act of June 28, 2006 on the sustainable management of radioactive materials and wastes. 20
Safety of the population and protection of the environment are set by a national framework law and are of the highest priority in determining disposal pathways for waste. Forecasts and inventories of waste lead to a National Management Plan, which is used to identify disposition pathways for all types of waste.
There is an effort to identify a safe disposition pathway proportionate to the hazard for each type of waste. French regulations do not allow for clearance of wastes from nuclear-related activities. France uses a policy of “waste zoning” at the generator's plant to segregate waste from zones that generate radioactive waste from those that do not.
The French radioactive waste classification scheme is shown in Figure 3-4 and described below:
Classification of radioactive waste streams in France. NOTES: Bq/g=becquerel per gram, CIGEO=Cigéo Project, CIRES= Centre industriel de regroupement, d'entreposage et de stockage facility, CSA= Centres de stockage de l'Aube, CSM=Centre de la Manche, (more...)
- Intermediate-level and low-level wastes are generated by the day-to-day operations at the nuclear power plants (NPP; green box in Figure 3-4 ). These wastes, previously disposed of at the Centre de la Manche disposal facility (CSM), are currently being sent to the Centres de stockage de l'Aube (CSA), which has been operational since 1992.
- Intermediate-level and high-level wastes are generated during uranium fuel recycling (i.e., reprocessing) (pink box in Figure 3-4 ). This waste will be stored in the geological disposal facility, the Cigéo Project. 21
- Very low-level waste is generated from shut-down and decommissioning (or dismantling) operations. This waste is disposed of at the Centre Industriel de Regroupement, d'Entreposage et de Stockage (CIRES) facility (upper blue box in Figure 3-4 ).
- Low-level, but long-lived, waste, is generated from graphite gas-cooled reactors and, for example, from the production of rare earth metals (lower solid blue box in Figure 3-4 ).
Waste from small producers or other nuclear activities can span the range of waste types shown in Figure 3-4 but represents a minor part of the inventory.
There are two characteristics shown in Figure 3-4 : activity levels and half-lives. Activity levels (rows in Figure 3-4 ) span orders of magnitude (less than 100 becquerels per gram [Bq/g] to more than 1 billion Bq/g) because there are specific threshold values for each radionuclide. Activity levels for very low-level waste range from 0 to 100 Bq/g with an average value of approximately 10 Bq/g. Waste is classified as “short-lived” or “long-lived” based on whether its half-life is less than or equal to or greater than 31 years, respectively (columns in Figure 3-4 ). The 31-year half-life is approximately the half-life of cesium-137, which is 30.17 years. 22
It is not possible from an operational standpoint to separate short-lived and long-lived radionuclides in NPP waste. There are always some long-lived radionuclides in this waste. WAC for very low-level and low-level disposal facilities in France allow for the disposal of waste containing certain amounts of long-lived radionuclides.
The principles behind radioactive waste disposal in France are, first, to contain and isolate the waste until it reaches a level of activity that does not represent significant hazard to the public or the environment (the monitoring phase in Figure 3-5 ). And, second, to limit the transfer of waste to the biosphere and to humans (the post-monitoring phase in Figure 3-5 ). As seen in Figure 3-5 , the containment phase lasts for about 300 years for near-surface disposal of waste with low levels of activity and several hundreds of thousands of years for geological disposal of high-level waste.
Disposal principles in the French radioactive waste management system. SOURCE: Gérald Ouzounian, ANDRA.
Dr. Ouzounian described the CSA disposal facility for low-level and intermediate-level short-lived waste. The facility was licensed and commissioned in 1992 with a total capacity of 1 million cubic meters—enough capacity to contain all of the low- and intermediate-level radioactive waste generated by the present fleet of French NPPs (58 reactors). The CSA facility was designed to contain and isolate the waste for 300 years, as required by the monitoring requirement mentioned previously, and to meet the requirements for the long-term post-monitoring phase.
The French waste disposal system employs the “defense-in-depth” concept with a multi-barrier system. The system consists of the waste package, which includes a containment material enveloping the waste (the first barrier); the disposal vault, which includes a network control gallery to control water that may flow through the disposal facility and final cover (the second barrier); and the geological environment, which has natural barriers such as clay to retard waste migration (the third barrier). This third barrier is the most important barrier in the post-monitoring phase.
Figure 3-6 is a schematic of the defense-in-depth disposal concept. A draining layer underlays the disposal facility, which in turn is underlain by an impermeable layer. The water table is shown with an outlet, labeled as “source” in the figure.
The French near-surface radioactive waste disposal concept. SOURCE: Gérald Ouzounian, ANDRA.
Inventory monitoring is essential for the effective management of radioactive waste—especially for managing long-lived radionuclides such as carbon-14, chlorine-36, and some beta emitters. NPP operators do not generally monitor for these isotopes because they do not impact daily plant operations. Therefore, the French regulator has established specific characterization requirements for these radionuclides for disposal purposes. For near-surface waste disposal, long-lived radionuclides are the major contributors to public doses in the post-monitoring phase.
Dr. Ouzounian's presentation also introduced France's approach to safety assessments, details on waste control acceptance criteria, and examples highlighting key aspects of safe operations and the defense-in-depth concept. Of particular relevance to this workshop was a discussion on the WAC for waste packages. These include:
- Radiological content
- Physical characteristics
- Chemical stability
- Gas generation
- Expected performance for long time periods
- Leaching rate
- Uniform distribution within the waste package (no hot spots)
Dr. Ouzounian provided historical perspective on the progression of safety rules, disposal concepts, and protection criteria in France. The safety rules were defined progressively, learning through the operational experiences of disposal facilities. Documents were updated and improved according to the experience of the operators—not the regulatory body. However, any changes to improve the safety rules are validated and endorsed by the regulatory body. General operational rules, and safety and radiation protection criteria, are also updated continuously.
John Applegate, the planning committee chair and executive vice president for University Academic Affairs of Indiana University, asked where the WAC (bulleted list above) came from and whether they had a risk basis. Dr. Ouzounian noted that the WAC were generated from safety assessments. Mr. Applegate also commented that experience at the prior disposal facility (CSM) appeared to be very helpful in designing the new facility (CSA), to which Dr. Ouzounian strongly agreed. All the incidents and malfunctions that occurred with the first disposal facility—which was designed without the benefit of detailed computer models—allowed for improvements to the new facility. The first safety regulations (1984 and 1985) are the result of the experiences from the first facility.
Dr. Ouzounian also noted the importance of adapting to knowledge gained from waste disposal experience in general. The process of developing an approach for the management and disposition of nuclear waste began in 1969, and much has been learned progressively. For example, it is now clear that the physical processes likely to occur should be well-understood and well-described, which requires high-quality modeling due to the long timescales involved. It is not possible to run an experiment for 100 to 300 years (or longer) to determine what may happen. The values, characteristics, and sources of hazards that are used in our assessments are the result of the models. This is why waste disposition decisions are site-specific, and also why we cannot transpose from one site to the other.
Dr. Robbins asked for clarification on one aspect of the French waste classification scheme. Is the irradiated graphite shown in Figure 3-4 considered LLW or intermediate-level waste according to the French classification scheme? Dr. Ouzounian explained that it is considered to be low-level but long-lived radioactive waste. One of the disposal options being studied is to segregate different types of graphite for disposal in different types of facilities depending on its irradiation level and activity.
3.3. DISCUSSION: KEY CHARACTERISTICS OF LLW AND CHALLENGING LLW STREAMS
Workshop chair John Applegate moderated the closing discussion on the first day's presentations. He noted that three organizing elements for managing challenging LLW streams were discussed:
- Characteristics of the waste. Defining waste characteristics is a technical issue. Mr. Applegate suggested that one could identify which characteristics are most important for making LLW disposal decisions. Alternatively, one could identify which characteristics are not important and are unnecessarily complicating waste disposal decisions.
- Waste management practices. Mr. Applegate asked whether participants could identify management practices that were unnecessarily slowing waste management decisions.
- Regulatory framework. Mr. Applegate asked participants to identify aspects of the current U.S. regulatory framework that are perceived to be failing. What can we learn from the experiences of other nations and international bodies? Mr. Applegate noted that regulatory flexibility is seen to be both useful as well as problematic. How do we manage that flexibility to make it useful, particularly with respect to increasing the predictability of the regulatory framework and/or eliminating requirements that aren't helpful?
Flexibility as a Double-Edged Sword
Kevin Crowley, director of the Nuclear and Radiation Studies Board at the National Academies, suggested that diversity and flexibility within disposal decision making is a double-edged sword. There is not much trouble handling diversity and flexibility from a technical standpoint. Where decision makers tend to fail is when they try to explain the diverse and flexible process to the people they serve. Dr. Crowley noted the importance of clearly communicating with the people who are served about the decision process: say what you are going to do, and do what you say you are going to do. Clear communication may be difficult when a system is too flexible and diverse.
Dr. Ouzounian argued that flexibility is crucially important, but it cannot be “free” flexibility. The flexibility needs to exist within a regulatory framework with clear rules, and one must be able to demonstrate that alternatives are safe and effective.
Mr. Applegate asked what a diverse and flexible framework might look like for LLW management. Mr. Garamszeghy responded that there are probably a couple approaches for establishing such a framework. One might use a performance standard, which requires a demonstration of how waste containment will be achieved. As long as the site is operated within an approved performance standard, there would be flexibility to make disposal decisions that meet that standard. This would be more flexible than a system that is based on compounding and conflicting regulations on allowable disposal options by waste type. Mr. Garamszeghy acknowledged that detailed regulations provide additional guidance to the user, but they also make it difficult to find innovative solutions when exceptions are presented.
Paul Black, chief executive officer of Neptune and Company, Inc., noted that although flexibility is critically important, cost-benefit analysis should also be considered in regulatory decisions and discussions. The current U.S. regulatory framework limits flexibility in strange ways because of competing regulatory structures. In order for the structure to change for the better, Dr. Black argued, one should strive for regulations that are simple and guidance that is process-oriented (rather than prescriptive) and based on cost-benefit considerations. The U.S. Office of Management and Budget (OMB) has the responsibility to evaluate new policies and rulemakings. As part of that evaluation, a cost-benefit analysis must be performed. OMB has developed guidance on using cost-benefit analysis. 23 Dr. Black suggested that both DOE and the USNRC should consider this guidance.
Mr. Applegate offered ALARA 24 as an example of a cost-benefit construct. Dr. Black strongly agreed and suggested that sustainability is another example. Sustainability balances three pillars: costs/economics, sociopolitical factors, and environmental factors. Dr. Black suggested that a framework for regulatory decision-making should combine the sustainability context ( National Research Council, 2011b ) with OMB's approach and guidance. Dr. Ouzounian noted that before cost-benefit can be assessed, safety must first be robustly demonstrated with a defense-in-depth approach.
Jennifer Heimberg, rapporteur and National Academies staff, asked Mr. Lovato whether he found it beneficial to have flexibility with the way DOE regulates over the USNRC's approach. She asked for any specific examples that showed how DOE's flexibility was utilized. Mr. Lovato noted that the NNSS does not have advance information about the variety of waste streams that will require disposal, so the DOE Orders are a good management structure for evaluating different types of waste streams. As an example, he cited radioisotope thermoelectric generators (strontium-90 sources originally from the Air Force) that required disposal. This waste had to be evaluated slightly differently from other waste streams; the flexibility in the DOE Orders allowed for that. However, he noted that it is always helpful to have a framework (e.g., the USNRC waste classification system) that can be used to explain waste management decisions to members of the public. Mr. Lovato was not advocating that a USNRC framework be used for DOE waste, but he cited it as the type of framework that is helpful for discussions with the public.
Elevating the Importance of Site Characteristics
Mr. Garamszeghy previously suggested that performance assessments be used as a framework for allowing flexibility in decisions while also providing boundaries. Mr. Applegate took this idea a step further by suggesting the following: One of the criticisms of the current U.S. regulatory framework is that it focuses on waste sources. What if the framework instead focused on disposal facilities? In other words, disposal decisions would be based on whether the waste could be safely disposed of in a facility as demonstrated by a performance assessment, irrespective of the waste source. For example, for waste potentially being sent to WCS, one would ask, “What does it take to make it safe there?”
Mr. Shrum supported this idea and restated it in another form: “Consider the waste. It can go here. It can't go there.” He noted that performance assessments have been done at all of the U.S. disposal facilities and is required under 10 CFR Part 61. But Mr. Shrum noted a potential communication problem with this approach: those whom we serve do not necessarily understand the details of a performance assessment, so they will not necessarily trust the output of the analysis. He said that the members of the public often do not understand that performance assessments are used to guide—not make—decisions. He supported Mr. Applegate's approach, but he noted that effective ways would need to be developed to educate the public for this approach to be successful.
He also noted that scientific understanding of radioactive wastes and disposal facilities have grown significantly since the 1950s, when commercial radioactive wastes were first disposed of. Mr. Shrum argued that this new understanding must be used to inform current disposal decisions. The nuclear industry as a whole has not been very good at describing the technical rationale for disposal decisions to the public, and, Mr. Shrum believes, that will have to change as part of a new framework.
Dr. Crowley noted that the workshop was intended to focus on exceptions. There are many exceptions to the existing regulations and rules, and there are questions about the best way to handle exceptions in the future. One option is to change the rules to include the exceptions. But this is unlikely in the short term. Another option is to establish procedures to handle the exceptions, for example by establishing “mini rules” that may not be incorporated into the regulations. Those mini-rules could be implemented at disposal facilities using their WAC, which of course are based on performance assessments.
However, it is difficult to anticipate the full variety of wastes that might come to a facility during its design or construction stages. On the other hand, one could probably think about unanticipated wastes during the design and construction stages and determine how they might be handled. Facility-specific performance assessments are a reasonable way to proceed.
Mr. Applegate commented that Dr. Crowley appeared to have endorsed his idea of focusing on disposal facilities instead of the waste source. A disposal facility could develop WAC to which waste streams are matched. Dr. Crowley agreed that this approach could work as long as the analysis was done within the framework of the current regulations. A near-surface disposal facility is only going to take certain types of waste; the framework suggested by Mr. Applegate should not be used to try to dispose of highly radioactive waste in near-surface facilities.
Dr. Black disagreed with the approach suggested by Mr. Applegate, primarily because he is not content with current regulations for radioactive waste disposal. They are overly conservative, so WACs developed using the existing regulations will also be overly constraining. For example, the inadvertent intrusion scenario in the regulations makes no sense for arid disposal sites according to Dr. Black.
Several years ago, Dr. Black developed a performance assessment for the Nevada Test Site (now NNSS), which allowed a user to enter the characteristics of a waste stream and get an answer within hours on whether it could be disposed of at the site ( DOE, 2006 and Crowe et al., 2005 ). Dr. Black argued that this is a better approach than WACs for evaluating whether a waste stream can be disposed of in a particular facility.
Taking Advantage of Knowledge Gained
Mr. Shrum previously introduced the idea of taking advantage of knowledge gained over decades of disposal operations, and Dr. Ouzounian also mentioned this idea in his case study. Scott Kirk, director of regulatory affairs at BWXT, raised this issue for further discussion, noting that the nuclear waste disposal industry has matured over the past 40 years. Modern state-of-the-art disposal facilities such as the WCS facility in Texas are remarkably different in siting and design than older disposal facilities such as Barnwell, which was state of the art in 1969. The modern sites are in arid environments, far removed from water tables, and designed with insights from 40 years of operating experience. These modern sites might be suitable for disposal of challenging LLW waste streams that could not be disposed of in older facilities. It would be useful to assess the suitability of current regulatory requirements against these modern facilities.
Charles Maguire, drector of the Radioactive Materials Division within the Texas Commission on Environmental Quality, highlighted the current state of regulations through an analogy. Most of the huge gothic cathedrals in Europe took approximately four generations to build. The last generation to work on the cathedral had little understanding of the reasons for the size, shape, or composition of the cornerstone. Yet the cathedral was built on it, and the generations of workers that followed improved their skills as cathedral construction progressed. Mr. Maguire noted that we are about to pass our nuclear knowledge on to a fourth generation of workers. But we are telling these workers to use the same tools and techniques as previous generations. We are not “getting better.”
Mr. Maguire asserted that we have to get better and to apply what we learn. We now take without question what the generation before said was essential, and we do not apply what has been learned about mitigating risk. He concluded that we need to make sure that as we build up the structure it becomes more beautiful or practical and that we are on a path to do better. Otherwise, we may end up with a regulatory framework that no one can afford to use.
From the Outside Looking In: Public Perception
Ms. Edwards suggested that terminology is important in communicating with the public, and that the LLW classification system makes clear communications difficult. Previously, one could refer to Class A LLW as a hazard that lasted about 100 years, Class C waste as a hazard that lasted 500 years, and high-level waste as a hazard that lasted tens of thousands of years. This hazard differentiation is important because the public can become confused between high-level and low-level waste. But the 1,000-year compliance period for certain types of LLW in the proposed 10 CFR Part 61 regulation blurs the previous hazard distinctions.
Mr. Camper noted that USNRC staff were trying to address the disposal of large amounts of depleted uranium and used this opportunity to add a requirement that was not previously embodied in the regulation (but should have been). The existing 10 CFR Part 61 does not specify a period of compliance but the proposed 10 CFR Part 61 rulemaking specifies a two-tiered approach to a period of compliance, i.e., Tier 1 at 1,000 years and Tier 2 up to 10,000 years.
Mr. Garamszeghy noted that the public perceives “nuclear” and “waste” as highly dangerous in part because of the complicated and prescriptive regulations that govern them. The thought is, “It must be dangerous because there are all these regulations to protect us.”
Mr. Applegate asked Mr. Garamszeghy to expand on his presentation about compensating the communities in which the Port Hope and Port Granby LLW facilities were sited. Was there a “general sense of fairness” argument? Or was it seen as compensating for risk? Or simply paying for the privilege? Mr. Garamszeghy explained that the intent of the PVP program was never to, for lack of a better word, “buy” public support. Rather, it was recognized that building and operating the LLW facility would strain the local communities in terms of a number of new people coming in and wear and tear on public facilities, for example. The PVP program ensured that the local towns, communities, and people were no worse off after the facility was in place than they would be if the facility was not there.
Dr. Crowley commented on the recurring topic of public perceptions and communications. The term “educating the public” is often used. He finds this term to be denigrating because it suggests that the public is not educated and that, if it were, the public would agree with the experts' conclusions—which is not always the case. Two-way communications are required to understand the concerns that the people who live around sites have about those sites.
Dr. Ouzounian noted that the term “stakeholders” is no longer used in France. Rather, the terms “concerned” or “interested parties” are used because this involves all parties, including waste producers and academics.
He also noted that the French Parliament passed a law in July 2016 as the result of a public debate on social benefits and responsibilities. The current generation benefits from the electricity generated by nuclear power plants, so it should be responsible for solving the waste management problem for following generations. The law required that a master plan describing all the major milestones of the lifetime of each disposal facility be developed and periodically reviewed. Initially, the planned review period was 10 years. However, Parliament decided that reviews will occur every 5 years with the involvement of all concerned or interested parties.
Dr. Ouzounian also commented on compensation to local communities. Compensation is provided because of expected damage to the infrastructure and the environment, resulting for example from large numbers of trucks on the roads during construction, not from increased risk. Parliament had another important debate in 2006. One side was arguing that nuclear industries were “buying the public” by giving money to communities. The other side was argued by the high commissioner for nuclear power in France. He pointed out that one community will accept the waste that belongs to all French people benefitting from electricity. This one community shows their solidarity with the country. He argued that, therefore, it was the responsibility of the rest of France to also show solidarity by supporting the community in developing its territory and its activities. This latter argument was accepted by the Parliament and ended comments about “buying the people.”
Dr. Black also commented on communication and public perception. He recalled that Mr. Shrum said that issues with LLW are more political than technical. The politics really come down to stakeholders, which means everyone associated with the disposal facility or the potential facility and the affected communities. The different outcomes for the Yucca Mountain and WIPP facilities provide a good example. In both cases, decisions on facility siting and construction were influenced by stakeholders and the political environment rather than the technical analyses. Dr. Black believes it is important to understand and “get on top of” the stakeholder issues before addressing regulatory change.
Mr. Camper spoke about the evolution of stakeholder engagement on USNRC decisions. Earlier in his career at the USNRC, staff would create new regulations and guidance documents without public input. But that changed over time for a number of reasons, not the least of which were regulatory failures. Stakeholders and interested parties demanded that decisions not be based entirely on the USNRC's scientific analyses. These demands have changed the way new regulations are developed and released.
“Regulatory Morass” Redux
Dr. Black commented that the “regulatory morass” that he referred to previously includes TRU waste. Defense TRU waste must be disposed of at WIPP, a deep geologic repository, but commercial waste containing less than 100 nCi/g of TRU nuclides can be disposed of in a near-surface disposal facility meeting the requirements of 10 CFR Part 61. Also, there are multiple regulations from DOE, USNRC, EPA, and the states for disposal facilities, some of which overlap or are in conflict.
“Challenging LLW streams” are defined as LLW streams that have potentially non-optimal or unclear disposition pathways due to their origin or content and incompatibility with existing standards, orders, or regulations.
REDOX (reduction oxidation) and PUREX (Plutonium and Uranium Recovery by Extraction) are processes for separating uranium and/or plutonium from irradiated fuel and targets.
In the 1950s, KAPL was a government research laboratory created by the U.S. Atomic Energy Commission (a predecessor agency to DOE).
“EPA: Defining Hazardous Waste: Listed, Characteristic and Mixed Radiological Wastes,” accessed February 25, 2017, https://www .epa.gov/hw /defining-hazardous-waste-listed-characteristic-and-mixed-radiological-wastes#character .
TCLP testing determines the mobility of organic and inorganic chemical species within in liquid, solid, and multiphasic wastes. TCLP testing follows specific guidelines established by EPA.
DOE had an existing contract with WCS, and WCS allowed for a shorter waste profile review time.
“Waste profiles” are required documents for shipping and acceptance of waste. The waste generator must submit a waste profile of each waste package for approval by the disposal facility prior to shipment. The disposal facility reviews the waste profiles to confirm the waste is compliant with the WAC of the disposal site.
“Application for License to Authorize Near Surface Disposal of Low-level Radioactive Waste, Appendix 5.2-1: Waste Acceptance Plan Revision 9,” see Section 5.2: Waste Profile Approval, accessed February 25, 2017, http://www .wcstexas.com /wp-content/uploads /2016/01/Waste-Acceptance-Plan.pdf .
In 2010, the sludge was consolidated into a single tank, the 509E tank, in preparation for waste processing and disposition.
Mrs. Hurley noted that, at the same time the liners were temporarily stored there, deactivation activities were also taking place to prepare for demolition of the G2 building.
The schedule accounted for the fact that concrete would not fully cure during the winter months (the SPRU tanks were covered by an unheated processing tent).
HEPA is the acronym for high-efficiency particulate air.
LLW containing hazardous chemicals is referred to as “mixed LLW.”
DOE defines “classified waste” in Order 435.1 as ( DOE, 1999, p. I-2 ): “Radioactive waste to which access has been limited for national security reasons and cannot be declassified shall be managed in accordance with the requirements of DOE 5632.1C, Protection and Control of Safeguards and Security Interests , and DOE 5633.3B, Control and Accountability of Nuclear Materials .”
CANDU refers to CANada Deuterium Uranium reactors. For more information, see: “Canadian Nuclear Association: CANDU Technology,” accessed February 25, 2017, https://cna .ca/technology /energy/candu-technology/ .
“Canadian Nuclear Association: Research Reactors,” accessed February 25, 2017, https://cna .ca/technology /research-development /research-reactors/ .
Mr. Garamszeghy identified one exception as some uranium mines in Saskatchewan, which has a dual federal-provincial regulatory framework.
There is a large contingent of weekend cottage owners in the area. When the poll was conducted, both full-time and part-time homeowners were contacted.
Note: the most recent update on this process was posted on April 15, 2017. The public comment period was closed on March 7, 2017. On April 5, 2017, CEAA requested additional information from OPG. “CEAA: Deep Geologic Repository Project for Low and Intermediate Level Radioactive Waste,” accessed April 27, 2017, http://www .ceaa-acee .gc.ca/050/details-eng .cfm?evaluation=17520 .
“ANDRA: Overview of national policy concerning radioactive waste management,” accessed February 25, 2017, http://www .andra.fr/international /pages/en /menu21/national-framework /overview-of-national-policy-1593.html .
France has made progress toward addressing its intermediate- and high-level wastes through the Cigéo Project, constructed in a clay formation at 500 meters depth and expected to be commissioned by 2025.
The Planning Act of June 28, 2006 on the sustainable management of radioactive materials and waste specifies that the half-life cut-off between short-lived and long-lived waste is 31 years.
“Circular A-4: Regulatory Impact Analysis: A Primer,” accessed March 27, 2017, https: //obamawhitehouse .archives.gov/sites /default/files/omb /inforeg/regpol/circular-a-4 _regulatory-impact-analysis-a-primer.pdf . Circular A-4 is referenced in the Trump administration's interim guidance: https://www .whitehouse .gov/the-press-office /2017/02/02/interim-guidanceimplementing-section-2-executive-order-january-30-2017 .
ALARA is “as low as reasonably achievable” and refers to the practice of reducing exposure to ionizing radiation through every reasonable effort. “USNRC: ALARA,” accessed February 25, 2017, https://www .nrc.gov/reading-rm /basic-ref/glossary/alara.html .
- Cite this Page National Academies of Sciences, Engineering, and Medicine; Division on Earth and Life Studies; Nuclear and Radiation Studies Board; Planning Committee on Low-Level Radioactive Waste Management and Disposition: A Workshop. Low-Level Radioactive Waste Management and Disposition: Proceedings of a Workshop. Washington (DC): National Academies Press (US); 2017 Apr 13. 3, Successful Disposition Case Studies.
- PDF version of this title (3.8M)
In this Page
- UNITED STATES CASE STUDIES
- INTERNATIONAL CASE STUDIES
- DISCUSSION: KEY CHARACTERISTICS OF LLW AND CHALLENGING LLW STREAMS
Recent Activity
- Successful Disposition Case Studies - Low-Level Radioactive Waste Management and... Successful Disposition Case Studies - Low-Level Radioactive Waste Management and Disposition
Your browsing activity is empty.
Activity recording is turned off.
Turn recording back on
Connect with NLM
National Library of Medicine 8600 Rockville Pike Bethesda, MD 20894
Web Policies FOIA HHS Vulnerability Disclosure
Help Accessibility Careers
- Utility Menu

Harvard GSAS Science Policy Group
Connecting policy & science in the graduate student community.

Looking for a Trash Can: Nuclear waste management in the United States

by Madeleine Jennewein figures by Rebecca Senft
Across the United States, nuclear waste is accumulating in poorly maintained piles. 90,000 metric tons of nuclear waste requiring disposal are currently in temporary storage. The United States, however, has yet to construct a long-term storage solution for this waste, leaving the nuclear material vulnerable to extreme weather events such as hurricanes , rising sea levels , and wildfire .
Nuclear power will be an essential tool in climate change adaptation because it’s capable of producing massive amounts of energy without any carbon emissions. In fact, although nuclear power is expensive to build, the scale and potential of nuclear power represents the most efficient path to eliminate carbon emissions from energy production . The drawback? Nuclear waste. Dangerous for thousands of years, nuclear waste requires long-term solutions that shield it from living things, but the public pressure to build effective storage solutions just isn’t there.
What is high-level nuclear waste?
Nuclear waste is primarily a byproduct of nuclear energy generation ( Figure 1 ). Nuclear energy harnesses the intense heat released from nuclear fission, where unstable atoms (either uranium or plutonium) are split into smaller elements. This heat turns water into steam, which spins turbines to generate electric power. However, the radioactive byproducts of nuclear energy generation are incredibly damaging to living things because nuclear decay also releases smaller particles—protons, neutrons and electrons—that can tear through tissue and damage genetic material, leading to cancers and birth defects.

Because of these adverse effects, nuclear waste must be treated carefully. Low-level waste (such as tools that have been contaminated with radiation) typically emits very low levels of radiation that are typically on par with the radiation we absorb daily from the sun. High-level waste, however, including spent nuclear fuel and its byproducts, is searing hot and requires years of cooling plus thick metal shielding to prevent radioactivity release. Even after 10 years of decay, this waste could emit 100 times a fatal dose of radiation in one hour.
How can nuclear waste be stored?
Nuclear waste storage facilities need to be designed to protect the waste from theft, shield it from emitting radioactivity, prevent it from leaking into water or soil, insulate it from release by natural disaster, and hide it from future generations that may not understand its danger. The main risk of nuclear waste is water running through the sealed storage containers (dry casks) and carrying nuclear particles out of storage. With this in mind, the two primary options for storage are protected sites above ground and geological repositories underground ( Figure 2 ).

Commercial energy generation produces the majority of nuclear waste in the U.S., which remains stored above ground near each of the 99 commercial nuclear reactors scattered around the country. Nuclear waste is stored in pools to cool for many years, and some is moved to above-ground concrete casks. However, these storage solutions are temporary at best. This form of storage requires personnel to maintain the sites of disposal, to monitor leakage, and to check the temperature and radioactivity of waste. Because nuclear waste could be repurposed for weapons, these pools and casks require a security presence to prevent theft. Above-ground casks are also vulnerable to natural disasters such as earthquakes, flooding, and hurricanes that could overwhelm the storage sites. While nuclear waste disposal sites are designed to safely store waste for several years, they haven’t been built to a standard that would allow the waste to sit there for centuries without constant upkeep.
Longer-term storage might be possible underground. Within geological repositories, the choice is between storage that is retrievable by future generations (geological disposition) or a sealed site that can’t be reopened (geological disposal). Decisions about which path to take need to contend with many questions: Can we confidently commit societal resources to manage this waste for the future? Can we scientifically minimize the chances of the waste escaping? Can we ensure social and political support for the project?
Geological disposal greatly diminishes many of the risks of nuclear waste, securely placing the waste underground, away from water sources, hurricanes, and humans. If located in an arid or frozen area isolated from earthquakes, a geological repository would be virtually impenetrable, and would effectively shield radioactive material. Thus, permanent geological disposal is the main goal of most countries. Many countries, with the U.S., Sweden, and Finland leading the effort, have begun the arduous process of selecting a site that meets intense scientific and logistical standards. Scientific research ensures that whichever site is selected will protect humans and isolate waste. Given the 24,000-year half-life of plutonium, scientists aim to design containment mechanisms and choose sites that will remain safe and isolated for 100,000 years.
Yucca Mountain
For the past 40 years, Yucca Mountain, in an arid desert 100 miles from Las Vegas, Nevada, was on track to become the main site for storing the U.S.’s accumulated nuclear waste ( Figure 3 ). The area has little precipitation, so little water would seep into the mountain. The extremely dense volcanic rock of the mountain has small pores, preventing any water leakage through the rock. In addition, waste would be stored far above water sources in the mountain. These features would effectively shield the waste and prevent the release of radioactivity.

In 1987, Congress directed the Department of Energy (DOE) to develop a nuclear waste storage facility at Yucca Mountain. Funded by a tax on nuclear power companies, researchers vetted the site and designed a storage plan for the mountain. In 2002, the DOE concluded that Yucca Mountain was suitable, and in 2008 they submitted an application to the Nuclear Regulatory Commission (NRC), an independent agency tasked with protecting safety related to nuclear energy, to begin the construction process.
Many Nevadans felt that the DOE did not solicit their input in the process and that having a nuclear waste repository so close by would be dangerous. In 2010, pressured by Senator Harry Reid (D-NV) and the Obama administration, the DOE withdrew its application. In turn, Congress withdrew funding, grinding the process to a halt. Still, the resulting legislation allowed the audit review to go forward, and the NRC reported in 2015 that Yucca Mountain satisfies nearly all of the regulatory requirements.
Why is it so hard to store nuclear waste safely?
The science and policy issues of nuclear waste demonstrate the need for science-informed policy but also show the limits of dictating policy through scientific assessment alone. Nuclear waste storage is a societal challenge. There is intense opposition in almost every community near a potential waste site. From decades of secrecy, bureaucracy, and top-down decision making, Americans distrust those who control nuclear waste decisions. In the absence of community engagement, Americans don’t recognize the acute need for nuclear waste storage, and perceive such storage as fallible and dangerous.
However, there is a way forward. While the U.S. fought over Yucca Mountain, Finland has quietly selected, licensed, and begun construction on a nuclear waste disposal facility . Key to Finland’s success was active community involvement in site selection. Indeed, Obama’s Blue Ribbon Commission to study waste disposal in the U.S. concluded that nuclear waste disposal could only be successful with active community involvement. The commission advocated starting a new process of site selection, with community engagement at all stages.
While many fear nuclear waste facilities in their communities, it is not necessarily anathema to all. Nuclear waste storage facilities would guarantee a steady stream of jobs and money for the community. For example, the WIPP site , a repository for lower-level radioactive waste from defense sources maintains much community support because the site provides jobs and actively engages the community, promoting itself as vital to national defense.
Where do we go from here?
While nuclear power constitutes around 20% of the power sources in the United States , and could be critical to climate change adaptation , six states currently prohibit nuclear plant construction until a nuclear waste storage facility is built. Waste storage is the essential piece of the puzzle.
As of May 2018, the House of Representatives voted to restart the Yucca Mountain process . The Trump administration has been sympathetic to reopening Yucca Mountain, asking for $150 million, but was ultimately denied. It’s unclear whether a nuclear waste repository will be built in the near future, but it’s increasingly clear how necessary and how difficult the process will be.
Madeleine Jennewein is a fifth-year Ph.D. student in the Virology program at Harvard University.
For more information:
- A more detailed description about how nuclear power works from the SiTN blog
- More information about the regulation and location of nuclear waste disposal sites from the US Nuclear Regulatory Commission
- An article expanding on the challenges of designing storage strategies to last thousands of years
- More information about possible nuclear waste storage strategies Originally posted on SITN
View the discussion thread.
Blog posts by month
- March 2023 (1)
- September 2022 (3)
- December 2021 (1)
- October 2020 (4)
- May 2020 (2)
CRESP Consortium for Risk Evaluation with Stakeholder Participation
- Projects Overview
- Waste Processing & Special Nuclear Materials
- Remediation, Near Surface Disposal & Long-term Stewardship
- Nuclear Waste Management Policy & Strategy
- Stakeholder Engagement & Communication
- Member Institutions
- Management Board
- Key Faculty & Technical Staff
- Reviews & Reports Overview
- Oak Ridge Risk Review Project
- Hanford Risk Review Project
- 2024 Workshop on Nuclear-Environmental Engineering & Science Education
- Education Overview
- CRESP Early Career Program
- Presentations
- Student & Scholar Highlights
- Courses Archive
- Workshops Archive
- PRACOP Archive
- CRESP by the Numbers
- Uncertainty in Long-Term Planning Nuclear Waste Management: Case Study
January 7-8, 2008, Vanderbilt University, Nashville, TN
Watch video and listen to podcasts from “Uncertainty in Long Term Planning-Nuclear Waste Management, A Case Study.” The Jan. 7-8, 2008, symposium and celebration was held in honor Frank L. Parker, Distinguished Professor of Water Resources and Environmental Engineering at Vanderbilt University.
The conditions of nuclear waste management today were examined as a basis for exploring the potential paths forward over the mandated time period of 1 million years. Technical, programmatic, social, financial and natural risks over these time periods, as well as inter- and intra-generational responsibilities, are considered. The possible changes, both positive and negative, in medical knowledge, technology, society and environmental conditions are discussed. Speakers and additional participants with diverse technical, social, political, philosophical and national backgrounds propose potentially societally acceptable paths forward and the advantages and disadvantages of each of the paths.
Sponsored by: The Mason Foundation, The Garrick Foundation, and The Consortium for Risk Evaluation with Stakeholder Participation (CRESP).
- No categories
- Workshop on Risk Assessment and Safety Decision Making Under Uncertainty
- Performance Assessment Community of Practice (PACOP)
- Nuclear Integration Project (NIP) Workshop
- Cementitious Materials for Waste Treatment, Disposal, Remediation and Decommissioning Workshop
- The Real Obstacle to Site Completion: Credible Post Remediation Sustainable Protection at Contaminated Sites with Residual Waste
- Can Science Really Foster Better Public Policy Decisions? The Lessons of the CRESP Experience
- Responsive Science: Forging Regulatory Resolution at DOE Sites
CRESP — Consortium for Risk Evaluation with Stakeholder Participation • Contact • Acknowledgements & Disclaimer
© Vanderbilt University • Nashville, Tennessee 37240
Your Vanderbilt
- Current Students
- Faculty & Staff
- International Students
- Parents & Family
- Prospective Students
- Researchers
- Sports Fans
- Visitors & Neighbors
Connect with Vanderbilt
©2024 Vanderbilt University ·
Academia.edu no longer supports Internet Explorer.
To browse Academia.edu and the wider internet faster and more securely, please take a few seconds to upgrade your browser .
Enter the email address you signed up with and we'll email you a reset link.
- We're Hiring!
- Help Center
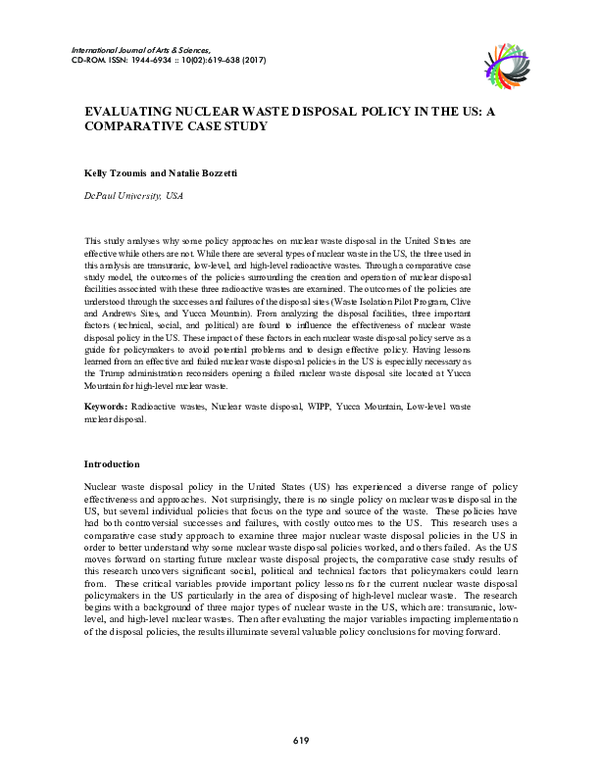
EVALUATING NUCLEAR WASTE DISPOSAL POLICY IN THE US: A COMPARATIVE CASE STUDY

This study analyses why some policy approaches on nuclear waste disposal in the United States are effective while others are not. While there are several types of nuclear waste in the US, the three used in this analysis are transuranic, low-level, and high-level radioactive wastes. Through a comparative case study model, the outcomes of the policies surrounding the creation and operation of nuclear disposal facilities associated with these three radioactive wastes are examined. The outcomes of the policies are understood through the successes and failures of the disposal sites (Waste Isolation Pilot Program, Clive and Andrews Sites, and Yucca Mountain). From analyzing the disposal facilities, three important factors (technical, social, and political) are found to influence the effectiveness of nuclear waste disposal policy in the US. These impact of these factors in each nuclear waste disposal policy serve as a guide for policymakers to avoid potential problems and to design effective policy. Having lessons learned from an effective and failed nuclear waste disposal policies in the US is especially necessary as the Trump administration reconsiders opening a failed nuclear waste disposal site located at Yucca Mountain for high-level nuclear waste.
Related Papers
Benjamin Sovacool
This article examines the problem of nuclear waste disposal and its implications for the future of the nuclear industry in the United States. Following a brief introduction, Part II investigates the process that generates spent nuclear fuel and discusses the current legal framework established by the Nuclear Waste Policy Act (NWPA). It also provides a brief overview of Yucca Mountain, succinctly tracing the project from its origins to the present. Part III summarizes developments in U.S. nuclear waste policy from 2002 to today, notably the creation of a Blue Ribbon Commission (BRC) on America’s Nuclear Future. Part IV discusses three of the central recommendations from the BRC’s final report. Part V analyzes available alternatives to Yucca Mountain and discusses the costs and benefits of each. Part VI provides conclusions pertaining to the sufficiency of the BRC’s recommendations and suggestions for policymakers. In following this structure, the article provides one of the first comprehensive critiques of the BRC’s suggestions and examines their effectiveness. Furthermore, it provides a necessary critical evaluation of the current statutory structure developed to address the nuclear waste disposal issue, and highlights major areas that policymakers must address if nuclear power is to have a future in the United States.
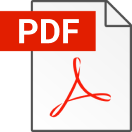
Michael Kraft
Naveen Srivatsav
Remediation Journal
Gordon Thompson
This paper reviews the history of the US effort to dispose of high-level radioactive waste created by operating nuclear fission reactors for military and commercial purposes. The history is considered here in three parts: the period beginning in 1957 and ending with passage of the Nuclear Waste Policy Act in 1982; implementation of that Act over the period 1982 to 2005; and recent plans to promote a nuclear power "renaissance", including the initiation of the Global Nuclear Energy Partnership in 2006. To date, disposal has not been accomplished, and many observers doubt that disposal will occur during the next several decades. The history of the disposal effort features a series of decision-action cycles in which objectives were formulated, decisions were taken, actions were implemented, and outcomes occurred. The decision-action process is examined here with attention to the roles and objectives of major stakeholders, the relation of those objectives to governmental decisions, and the extent to which the actual outcomes have corresponded with the objectives.
Policy Studies Journal
Energy Research & Social Science
Marissa Z . Bell
With continued reliance on nuclear energy in the United States, we are increasingly having to contend with the problem of nuclear waste. Growing quantities of spent nuclear fuel, the lack of permanent storage, and an increasing number of decommissioned nuclear reactors are some of the techno-economic and political realities that have thrust US nuclear waste management into a liminal space of uncertainty. Using a qualitative approach combining policy analysis and case studies, we examine this liminality and the consequent emergence of a new political economy of spent fuel management. Our findings indicate a series of regulatory, organizational and spatial 'fixes' have recently emerged to capitalize on the liminality of nuclear waste management in the US. These fixes include the US Nuclear Regulatory Commission's Continued Storage Rule, the proposal of private consolidated interim storage facilities, and the reconfiguration of nuclear waste management corporations into vertically integrated nuclear waste assemblages with private equity involvement. Such developments are potentially problematic in that they are inherently contradictory, temporary, and quick 'fixes' that do not solve the underlying societal issues, such as distrust in science and lack of democratic governance and energy justice in decision-making, and in many cases they delay permanent solutions. This work contributes to the fields of economic geography, science and technology studies, and nuclear policy studies, by analyzing novel corporate arrangements and developments in the management of spent nuclear fuel in the US. The findings suggest a need to carefully assess policy solutions across contexts, from nuclear waste management to other rapidly evolving energy sectors.
Susan Gawarecki
Review of Policy Research
Bruce Clary
Helen Neill
Scientists who work in emerging technologies focus on the advantages and are surprised by the sometimes negative responses from citizens who question the use of such technologies. Social scientists have explored the sources of this sometimes contentious debate in emerging technologies such as biotechnology and nanotechnology [1]. While radioactive waste disposal does not qualify as an em erging technology per se, response to Yucca Mountain as a proposed disposal site shows that tensions remain [2,3,4]. This paper identifies theories and empirical results from multiple literatures to explore and compare public responses to nanotechnology, biotechnology, and nuclear technologies. Several issues arise while exploring what is known about public response to each technology. Given the importance of nuclear waste management and disposal [5] for present and future generations, research fro m the social sciences may offer a more constructive approach to the substantive problems that may aris...
Loading Preview
Sorry, preview is currently unavailable. You can download the paper by clicking the button above.
RELATED PAPERS
Risk, Hazards & Crisis in Public Policy
Urban Strandberg
Symposium in the RISCOM Programme: Values in …
claire mays
Sarah Widder
Henry Mayer
Carol Silva
Hank Jenkins-Smith
Stephen Couch
Matthew Cotton
Journal of Nuclear Science and Technology
Tatsujiro Suzuki
Bret Leslie
Journal of Policy Analysis and Management
Dennis Coates
Risk Analysis
William Desvousges
Omar M Al-Qudah
Omar Al-Qudah
CAROL SILVA
Contemporary Sociology
Jeryl Mumpower
Anne Bergmans
Frank Perry
Lori A Cramer
Historical Social Research
Sophie Kuppler
Journal of Environmental Planning and Management
Karen Lowrie
Journal of Risk Research
RELATED TOPICS
- We're Hiring!
- Help Center
- Find new research papers in:
- Health Sciences
- Earth Sciences
- Cognitive Science
- Mathematics
- Computer Science
- Academia ©2024
Sustainable Heritage Case Studies
Carleton university student case studies, interweaving traditional knowledge in managing nuclear waste.
Indigenous Knowledge in Canada’s Nuclear Waste Management Organization
Case Study prepared by Nivethini Jekku Einkaran, Carleton University
Keywords: Nuclear Waste Management, Indigenous Knowledge, Communities, Policies, Engagement
LESSONS LEARNED
The Nuclear Waste Management Organization (NWMO) is responsible for the safe management and disposal of nuclear waste in Canada. The organization has taken up an inclusive, open and transparent participatory approach including interested communities and Indigenous Peoples, prioritizing safety and security of the people and environment in the process. The organization has incorporated a two-eyed approach in bridging the gap between Traditional and Western Sciences to value both equally. The NWMO is working on shared ethics identifying similarities and differences by acknowledging, recognizing, respecting, honoring and supporting Indigenous Knowledge, belief systems, culture, tradition, values, customs, stewardship, language of the Indigenous peoples. The organization is conducting workshops between technical staff and Indigenous peoples to help both understand/educate about each other. As a result of the constant engagement with Indigenous peoples, NWMO has formulated policies to govern the usage of Indigenous Knowledge / Traditional Ecological Knowledge in the nuclear waste management process. “The development of this policy has benefited from advice from the NWMO Council of Elders and Youth, and through our relationships with Aboriginal organizations at the national, provincial, and regional levels” ( Aboriginal Policy , 2009). Continuous revisitation of these policies, concerns and issues is helping in progress of engaging communities. NWMO has planned for a Deep Geological Repository as Canada’s approach to nuclear waste management after consultation with experts and communities.
(Note: The words ‘Aboriginal’, ‘Indigenous’ are used interchangeably in order to maintain the terms used in the references)
PRESENTATION
Preliminary research on the involvement of Indigenous communities in the decision-making process in managing nuclear waste in Canada was presented on November 21, 2019 in class. Major focus was given to the policies adopted by Nuclear Waste Management Organization i engaging Indigenous peoples in the process to safely store, manage and discard nuclear waste.
DESCRIPTION
The Nuclear Waste Management Organization (NWMO) is a non-profit organization established in 2002 under the Nuclear Fuel Waste Act (NFWA) by Canada’s nuclear electricity producers, Ontario Power Generation (OPG), New Brunswick Power Corporation and Hydro-Quebec (HQ) along with Atomic Energy of Canada Limited (AECL). The organization “is responsible for designing and implementing Canada’s plan for the safe, long-term management of used nuclear fuel” (NWMO, n.d.). The organization regards safety of the people and environment as the top priority in the process of used nuclear fuel management. They are guided by six values: Safety, Integrity, Excellence, Engagement, Accountability, Transparency (NWMO Mandate, n.d.). The government of Canada approved Adaptive Phased Management (APM) suggested by NWMO as Canada’s approach for long-term management of nuclear waste which is both a technical method and a management system. NWMO launched a nine-step process in 2010 and is currently in the site selection process.
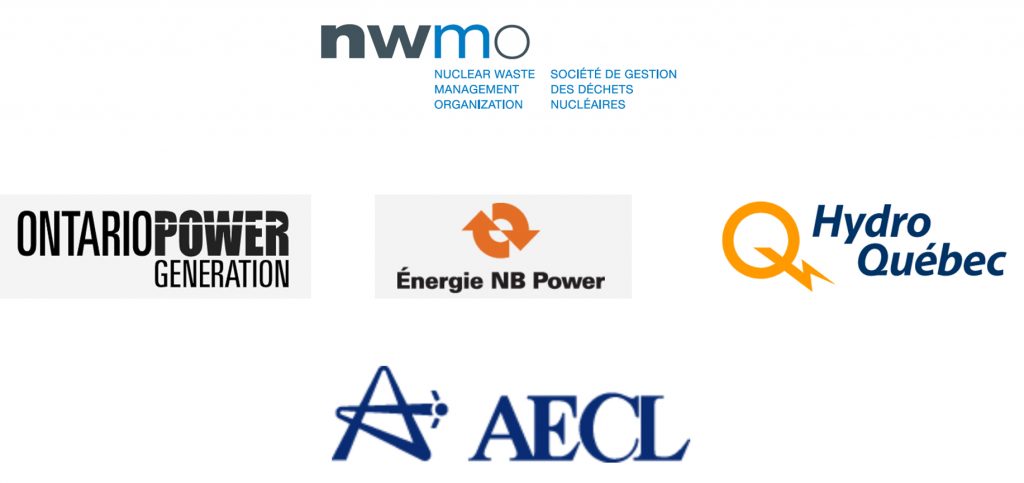
NWMO has been working closely with experts and the public, including First Nations, Métis and Inuit peoples since its establishment. “The NWMO is obliged under the NFWA to consult with Canadians and Aboriginal peoples throughout the implementation of Adaptive Phased Management (APM).” (NWMO, n.d.).
Aboriginal Policy , 2009 : This was the first policy adopted by the NWMO with respect to engagement with Indigenous Peoples and it focused on recognizing and respecting Aboriginal Peoples beliefs on environmental stewardship; acknowledging their unique status, rights and treaties; recognizing their unresolved land claims; building relationships on a foundation of respect for their language, cultural protocols, customs, political, social, economic and political institutions; pursuing agreements for the mutual benefit of all.
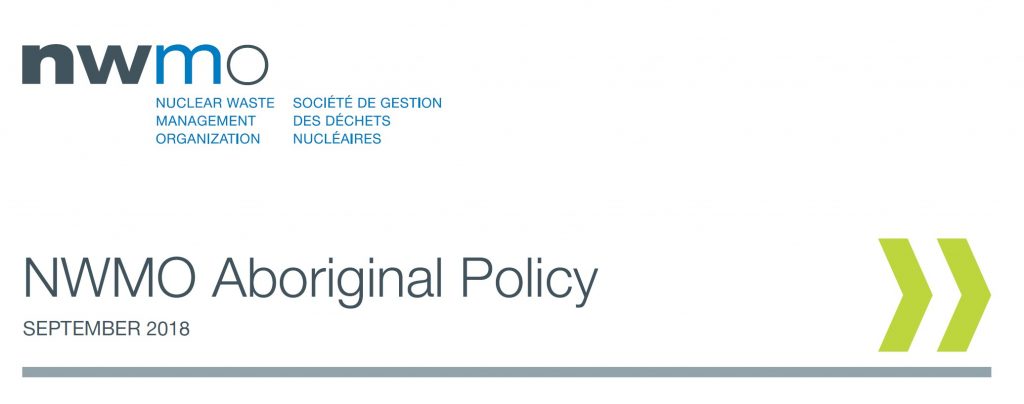
Indigenous Knowledge Policy , 2016 : This policy deals with the use of Indigenous Knowledge in the NWMO’s process for the development of a Deep Geological Repository. It commits that Indigenous Knowledge will inform decisions in the planning process by looking to share their Knowledge to the extent they wish while protecting Indigenous Intellectual property; recognizing the collection, documentation and managing of Indigenous Knowledge is carried out with the interest of Indigenous communities; entering into MOUs or agreements for the protection of Indigenous Knowledge, supporting Indigenous engagement; supporting the development of community steering committees by Indigenous peoples to oversee the work; continuing to seek high-level policy advice from the Council of Elders and Youth and Indigenous governments.
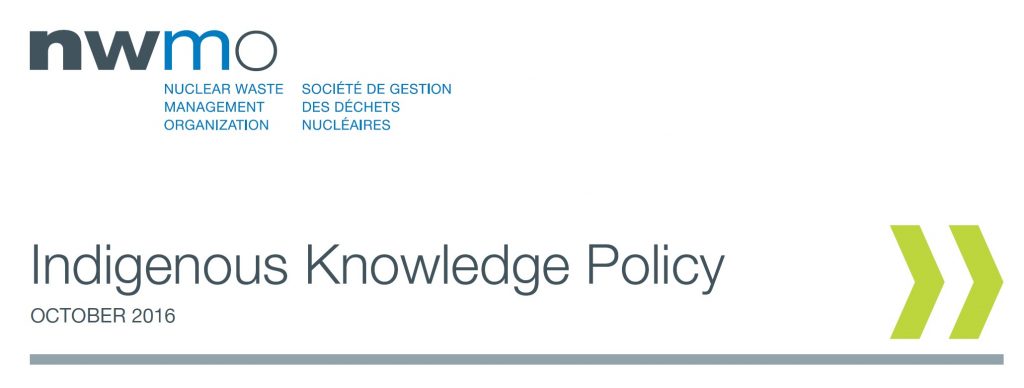
Reconciliation Statement, 2018 : NWMO released a Reconciliation Statement as a contribution to their reconciliation strategy upon the good track of maintaining Indigenous relations since its establishment as stated by Bob Watts, Vice-President of Indigenous Relations. It read
“In the context of reconciliation, the Nuclear Waste Management Organization (NWMO) recognizes historical wrongs in Canada’s past and the need to create a better future by addressing the challenges of today. The NWMO Council of Elders and Youth speaks of this journey as a new era for humanity – a time of reconciliation with First Nation, Métis and Inuit peoples. The NWMO is committed to contribute to reconciliation in all its work by co-creating a shared future built on rights, equity and well-being. In addition, the NWMO will establish a Reconciliation Policy with an implementation strategy that will be measured annually and publicly reported to contribute to the Truth and Reconciliation Commission’s calls to action.” Reconciliation Statement, 2018
Reconciliation Policy, 2019: With respect to the Reconciliation Statement and Truth and Reconciliation Commission’s Call to Action, the NWMO Reconciliation Policy was adopted in 2019. It built on the Aboriginal Policy and has now replaced it. The Reconciliation Policy focuses on committing to meaningful engagement and consultation, building respectful relationships, seeking the free, prior, and informed consent of impacted Indigenous Peoples; providing impacted First Nation and Métis people with equitable access to jobs, business opportunities, training and education in NWMO’s work; committing to provide education for management and staff on the history of Indigenous Peoples; working with impacted communities to implement the mandate and informing the site selection process with best available knowledge and ethics; working with Crown; committing to respecting and following local First Nation and Métis protocols related to burial sites found on work sites; develop an annual reconciliation plan; communicating clearly with new employees on the commitment to engagement with Indigenous peoples.
- Thousands of years ago – Indigenous Peoples started settling in Canada
- 1763 – Royal Proclamation
- 1871 to 1921 – Numbered treaties or Post-Confederation treaties, a series of eleven treaties were signed between First Nations and the reigning monarch
- 50 years ago – Canada started generating power from nuclear energy
- 1982 – Constitution Act – Section Thirty-five provides constitutional protection to the indigenous and treaty rights of indigenous peoples in Canada
- 1985 – Indian Act – Canadian act of Parliament that concerns registered Indians, their bands, and the system of Indian reserves
- 2007 – United Nations Declaration on Rights of Indigenous Peoples (UNDRIP)
- 2014 – Truth and Reconciliation Commission recommended 94 Calls to Action
The following table brings out the evolution of NWMO and its engagement with Indigenous peoples. (The table has been split for clarity.)
Nuclear Fuel Waste Act was passed Nuclear Waste Management Organization was established | Advisory Council includes Indigenous representation | |
Traditional knowledge workshop | ||
Adaptive Phased Management (APM) was proposed Ethical and Social Framework was adopted | Elders Forum was formed. Working group was named Niigani (meaning ‘leading the way’ | |
APM was accepted by the Canadian government as Canada’s long-term approach to manage nuclear waste | Board of Directors includes Indigenous representation | |
Traditional Knowledge Project (Personal experiences in Ontario’s DGR to educate the Indigenous peoples about the nuclear waste management process and in Mackenzie River to enable the technical staff to value Indigenous peoples; and a Workshop) | ||
Memorandum of Understanding between Natural Resources Canada (NRCan) and NWMO Funding formula approved by the Minister of Natural Resources | ||
NWMO launched a nine-step approach Siting process initiated | ||
Elders Forum renamed as Council of Elders | ||
Council of Elders adds Youth | ||
NWMO’s Director of Nuclear Design and Transportation Chris Hatton receives Innovation Achievement Award from Canadian Nuclear Society | ||
Declaration of the Keepers of the Land signed by Council of Elders and Youth | ||
Consultation on borehole drilling | ||
finalized | ||
issued |
STAKEHOLDERS
Organizations and governing authorities
- Nuclear Waste Management Organization
- Minister of Natural Resources
- Nuclear Fuel Waste Bureau
Agencies
Ontario Power Generation (OPG) New Brunswick Power Corporation (NB Power) Hydro Quebec (HQ) Atomic Energy of Canada Limited (AECL) | Canadian Nuclear Association (CNA) Canadian Nuclear Safety Commission (CNSC) Canadian Environmental Assessment Agency (CEAA) | Natural Resources Canada (NRCan) Environment Canada Fisheries and Oceans Canada Health Canada |
The funding agencies are required to maintain a trust to manage the funds. Effectively, it is the citizens who are contributing to the project as it is the money paid in the form of bills (NWMO, Funding, n.d.)
Communities : 22 communities (municipalities and Indigenous peoples) showed interest to host the process.
Currently the NWMO has narrowed the selection down to two potential siting areas. “The Township of Ignace in northwestern Ontario, and Township of Huron-Kinloss and Municipality of South Bruce in southern Ontario are considered potential host areas for the project” (NWMO, Site Selection, n.d.)
Indigenous Communities
Congress of Aboriginal Peoples (CAP) Inuit Tapiriit Kanatami (ITK) Métis National Council (MNC) Native Women’s Association of Canada (NWAC) Pauktuutit Inuit Women’s Association | Atlantic Policy Congress of First Nations Chiefs (APC) Eabametoong First Nation, Fort Hope, Ontario East Coast First People’s Alliance Federation of Saskatchwen Indian Nations Northern Saskatchwen Ontario Métis Aboriginal Association (Ontario affiliate of CAP) Sakitawak Métis Society, Northwestern Saskatchwen Union of New Brunswick Indians Western Indian Treaty Alliance (WITA) Prince Albert Grand Council |
International Partners: Nuclear Energy Agency (NEA) of the Organization for Economic Co-operation and Development (OECD); International Atomic Energy Agency (IAEA); NWMO has signed or renewed co-operation agreements with Nuclear power counterparts – Sweden, Switzerland, Finland, France, Korea, Japan, and the United Kingdom; as NWMO is committed to international knowledge sharing (NWMO, News Release, 2018). In addition, these countries have Deep Geological Repositories to discard nuclear waste.
Natural heritage: As mentioned in the Initial Screening criteria, NWMO has decided to avoid ecologically sensitive areas (protected areas) and areas of local significance (heritage sites, provincial parks, national parks) in the site selection process. In addition NWMO has committed to select a site where there are no sources of available groundwater at repository depth (of 500 m); where there are no economically exploitable natural resources available with the future generations in mind; which has no known geological and hydrogeological characteristics which could hinder the safety of the site (NWMO, Site Requirements, n.d.). As a result of this cautious approach, NWMO is preserving biodiversity in its commitment to safety and security. The Deep Geological Repository has been considered the safest mode of nuclear waste disposal internationally (NWMO, n.d.).
“Storage of radioactive waste has been demonstrated to be safe over some decades and can be relied upon to provide safety as long as active surveillance and maintenance is ensured. In contrast, geological disposal promises long term safety without surveillance and maintenance.” (IAEA, 2003)
Cultural heritage: Values, traditions, customs and rights of Indigenous Peoples, First Nations and Métis communities are being acknowledged, respected, recognized and supported in the sharing of Traditional Ecological Knowledge/Aboriginal Traditional Knowledge/Indigenous Knowledge in the process of site selection and in all future processes of NWMO (Traditional Knowledge Workshop, 2008). This can be regarded as the first step in bridging the gap between Indigenous knowledge and Western Sciences. NWMO through its policies and seeking advice and consensus from the Indigenous Communities, Council of Elders and Youth has aided in the sustenance of culture and social values of the Indigenous peoples.
Linking Natural and Cultural Heritage: Passing on heritage information to the distant future has been made possible through the proposal of Centre of Expertise (NWMO, Surface facilities, n.d.). By closely working with communities and Indigenous people, and experts, NWMO is addressing the same goals in different views. This aspect of linking natural and cultural heritage satisfies Target 11.4 which advocates for strengthening efforts to protect natural and cultural heritage under Sustainable Development Goal 11 on Sustainable Cities and Communities.
“The engineering challenge of creating storage that will last for this time, is dwarfed by the communication challenge of explaining what it is to people in the distant future. This combination of preservation and communication makes partnership between heritage management and nuclear waste management fruitful.” (Heritage Futures)
SUSTAINABILITY
Environmental sustainability: NWMOis committed to achieving livability through balanced growth with major focus on health and safety of the community and environment (NWMO, Mandate, n.d.). NWMO adopted an Environmental Policy that would guide all the processes. Environmental Assessment teams assess impacts on ecosystem and of climate change in the sites under consideration. NWMO states the reason for choosing a DGR as “A geological repository uses multiple barriers that include the waste form, container, sealing materials, and the host rock. The system is designed such that the failure of one component would not jeopardize the safety of the containment system as a whole.” OntarioPower Generation has a Deep Geological Repository near Lake Huron in Kincardine for the disposal of low-level and intermediate-level nuclear waste. The DGR proposed by NWMO will house the disposal of high-level waste (i.e.,) Used Nuclear Fuel (NWMO, Deep Geological Repository, n.d.).
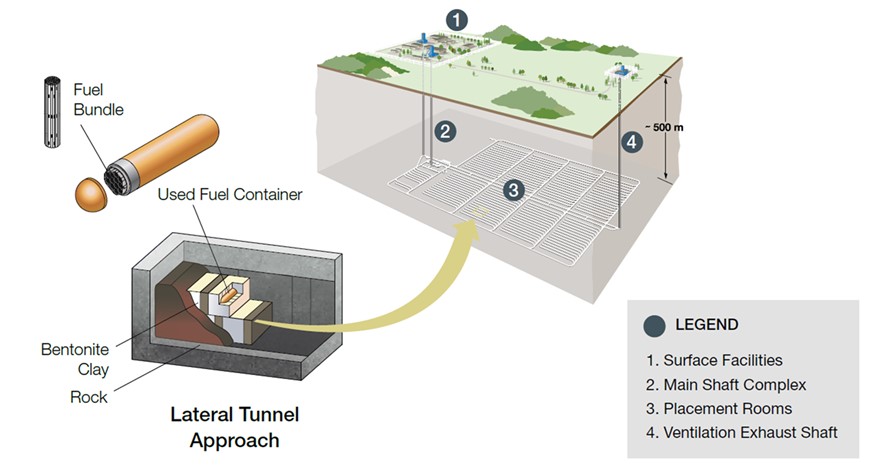
The NWMO works comply with Sustainable Development Goal 12 on Responsible Consumption and Production with focus on Targets 12.2 that deals with sustainable management and effective use of resources and Target 12.5 on waste reduction.
Socio-cultural sustainability: NWMOis working based on shared ethics with Indigenous peoples; and integrating Indigenous Knowledge in decision-making process which aids in the sustenance of their culture and social aspects. NWMO has plans for the construction of a Centre of Expertise at the site of the Deep Geological Repository to act as a place for testing and assessment of the site, and, sharing knowledge after the operations have begun and to convey about the work to the future generations (NWMO, Centre of Expertise, n.d.). This complies with Sustainable Development Goal 4 – Quality Education and specifically Target 4.7 which deals with the need for all learners to acquire the required skills to promote sustainable development. In addition, it also fulfills Targets 17.9 on capacity building in the implementation of goals and Target 17.17 on Multi-Stakeholder partnerships which involves engaging public, building on experience and resourcing strategies for partnerships, which are discussed under Sustainable Development Goal 17 on Partnership for the goals.
Economic sustainability: NWMO is funding involved communities as a form of recognition and is discussing with communities and Indigenous peoples on compensation of impacts – creating job opportunities is one among them as it has been stressed by the Indigenous communities in the workshops and dialogues held. Apart from creating jobs, NWMO currently has scholarships and donations programs for students from Indigenous, First Nations and Metis communities which satisfies Sustainable Development Goal 4 that deals with ensuring inclusive and equitable quality education.
MEASUREMENT
NWMO’s works for management and disposal of nuclear waste and their Indigenous relations as assessed based on four measurement tools:
United Nations Convention on Biological Diversity: The organization has adopted policies that applies to all works of NWMO with Indigenous peoples based on the United Nations Convention on Biological Diversity. Article 8(j), in particular, refers to indigenous knowledge and requires that: “The traditional knowledge of indigenous and local communities be respected, preserved and maintained; that the use of such knowledge should be promoted for wider application with the approval and involvement of the holders of such knowledge; and that they should equitably share in the benefits which arise from the use of their knowledge” (United Nations, 1992).
David Harmon in his article ‘A Bridge Over the Chasm’ has pointed out some of the factors to be considered in bridging natural and cultural heritage:
NWMO is working on the concept of shared ethics through its policies and continuous engagement with Indigenous communities. | |
NWMO works on the principles of safety and security of the people and the environment and Indigenous peoples refer to it as environmental stewardship. This common term of reference has enabled the continuous engagement of Indigenous peoples possible in the decision-making process ( 2009 and , 2019). | |
NWMO has taken up a community driven approach in safely managing and disposing nuclear waste apart from the policy or regulatory agencies, experts in nuclear power and environmental consultants. This interdisciplinary approach has ensured that everyone involved are well informed through the sharing of knowledge from both Traditional and Western sciences. | |
NWMO is organizing workshops to help in the exchange of knowledge to achieve best outcomes that are beneficial to everyone. The Traditional Knowledge Project of 2008 is a notable initiative that focused on personal experience gained by the Indigenous peoples on scientific approaches involved in a Deep Geological Repository through the visit to the DGR that was under construction in Kincardine, Ontario and the experience gained by technical staff to understand and value the culture and traditions of Indigenous peoples through the Cultural immersion in Traditional Knowledge on Mackenzie River. This project ended with a workshop to integrate both Traditional and Western sciences. |
Measuring NWMO’s Indigenous Relations with respect to United Nations Declaration on the Rights of Indigenous Peoples :
States…obtain their free, prior and informed consent before adopting and implementing legislative or administrative measures that may affect them. | The NWMO commits to…seeking the free, prior, and informed consent of impacted Indigenous peoples before proceeding with development of a deep geological repository. ( , 2019) |
States shall establish and implement, in conjunction with indigenous peoples concerned, a fair, independent, impartial, open and transparent process, giving due recognition to indigenous peoples’ laws, traditions, customs and land tenure systems, to recognize and adjudicate the rights of indigenous peoples pertaining to their lands, territories and resources, including those which were traditionally owned or otherwise occupied or used. Indigenous peoples shall have the right to participate in this process. | NWMO has taken up an inclusive, open and transparent approach engaging communities and Indigenous peoples in the decision-making process. The organization has a Council of Elders and Youth who are a part of the Advisory Council and guide the NWMO’s implementation process. Through the formulation of policies that guide the engagement of Indigenous peoples and sharing of Traditional Knowledge in the process, the organization ensures that the rights and concerns of the Indigenous communities are addressed, Indigenous Knowledge is protected in the sharing process which has encouraged their participation in the decision-making process. |
2. States shall take effective measures to ensure that no storage or disposal of hazardous materials shall take place in the lands or territories of indigenous peoples without their free, prior and informed consent. | The affirms that the implementation process of the Deep Geological Repository for the disposal of nuclear waste will seek the free, prior and informed consent of the Indigenous peoples. |
Ontario Nature has published Guiding Principles for Engagement in Conservation Offsetting with respect to Indigenous Communities:
“The NWMO commits to… seeking the free, prior, and informed consent of impacted Indigenous peoples before proceeding with development of a deep geological repository.” ( , 2019) | |
, 2019, “The NWMO commits to providing education for management and staff on the history of Indigenous peoples, …which will require skills-based training in intercultural competency, conflict resolution, human rights, and anti-racism as stated in the Truth and Reconciliation Commission call to action # 92.” “The NWMO commits to respecting and following local First Nation and Métis protocols related to burial sites found on work sites.” This commitment to contribute towards reconciliation by respecting Indigenous peoples, their rights, views and knowledge, and to pass it onto new employees has enabled the technical staff to value Indigenous peoples’ values and views. | |
NWMO is engaging communities and Indigenous peoples in the site selection process of the Deep Geological Repository to address the concerns and issues raised by them in terms of environmental and social impact. | |
NWMO is working on shared ethics to ensure equitable participation and cooperation in all the stages of the decision-making process. | |
NWMO is organizing workshops to inform the Indigenous peoples of the scientific approach and in their commitment to respect Indigenous languages, is publishing the reports (of the workshops and other details) in 13 different languages apart from English (NWMO, n.d.) to ensure best communication and maintain transparency in the process. |
Books/Book chapters/Journal articles
- Harmon, David, 2007, “A Bridge over the Chasm: Finding Ways to Achieve Integrated Natural and Cultural Heritage Conservation,” International Journal of Heritage Studies, 13. 4–5: 380–392.
- ON Nature magazine – Indigenous Perspectives on Conservation Offsetting. (2017, February). Retrieved from https://view.publitas.com/on-nature/indigenous-perspectives-on-conservation-offsetting.
- Barnaby, J., & Emery, A. (2008, November). Report of Traditional Knowledge Project. Retrieved from https://www.nwmo.ca/~/media/Site/Files/PDFs/2015/11/04/17/32/916_NWMODR-2008-03ReportofTraditionalKnowledgeProject.ashx?la=en.
- Implementing Adaptive Phased Management 2019 to 2023. (2019, March). Retrieved from https://www.nwmo.ca/~/media/Site/Reports/2019/03/19/14/45/NWMO-201923-Implementation-Plan–EN.ashx?la=en.
- Moving Forward Together: Process for Selecting a Site for Canada’s Deep Geological Repository for Used Nuclear Fuel. (2010, May). Retrieved from https://www.nwmo.ca/~/media/Site/Files/PDFs/2015/11/04/17/34/1545_processforselectingasiteforcan.ashx?la=en .
- INTERNATIONAL ATOMIC ENERGY AGENCY, VIENNA, (2003). The Long Term Storage of Radioactive Waste: Safety and Sustainability. Retrieved from https://www-pub.iaea.org/MTCD/Publications/PDF/LTS-RW_web.pdf
- Indigenous Knowledge Policy: NWMO. (2016, October). Retrieved from https://www.nwmo.ca/~/media/Site/Reports/2016/10/20/11/02/English_IndigenousKnowledgePolicy_2016.ashx?la=en .
- NWMO Aboriginal Policy. (2009)
- NWMO Environment Policy. (2018, January). Retrieved from https://www.nwmo.ca/~/media/Site/Files/PDFs/2019/05/16/17/25/EN_EnvironmentPolicy_2018.ashx?la=e n.
- Reconciliation Policy. (2019, October). Retrieved from https://www.nwmo.ca/~/media/Site/Files/PDFs/2019/10/17/14/55/Reconciliation-Policy-2019.ashx?la=en.
- United Nations. 2015. Transforming Our World: the 2030 Agenda for Sustainable Development. https://sustainabledevelopment.un.org/content/documents/21252030%20Agenda%20for%20Sustainable%20Development%20web.pdf
- United Nations. 2007. United Nations Declaration on the Rights of Indigenous Peoples. https://www.un.org/development/desa/indigenouspeoples/wp-content/uploads/sites/19/2018/11/UNDRIP_E_web.pdf
- United Nations. 1992. CONVENTION ON BIOLOGICAL DIVERSITY. https://www.cbd.int/doc/legal/cbd-en.pdf
News releases by the NWMO:
- The NWMO issues Reconciliation Policy, calls on corporate Canada to join discussion: NWMO. (2019, October). Retrieved from https://www.nwmo.ca/en/More-information/News-and-Activities/2019/10/16/15/03/The-NWMO-issues-Reconciliation-Policy-calls-on-corporate-Canada-to-join-discussion .
- The NWMO issues Reconciliation Statement collaboratively with Indigenous Elders and youth: NWMO. (2018, July). Retrieved from https://www.nwmo.ca/en/More-information/News-and-Activities/2018/07/16/16/47/The-NWMO-issues-reconciliation-statement-collaboratively-with-Indigenous-Elders-and-youth.
- The NWMO Signs Co-Operation Agreements with International Partners. (2018, May). Retrieved from https://www.nwmo.ca/en/More-information/News-and-Activities/2018/05/09/11/44/The-NWMO-Signs-Cooperation-Agreements-with-International-Partners .
Key sections of the NWMO website:
- Nuclear Waste Management Organization. (n.d.). https://www.nwmo.ca.
- 11. Aboriginal Dialogues: NWMO. (n.d.). Retrieved from https://www.nwmo.ca/en/Canadas-Plan/Selecting-APM-A-Three-Year-Study/The-Study/Dialogue-Reports/11-Aboriginal-Dialogues .
- A Collaborative Approach: NWMO. (n.d.). Retrieved from https://www.nwmo.ca/en/Canadas-Plan/About-Adaptive-Phased-Management-APM/Implementing-the-Plan/A-Collaborative-Approach.
- Council of Elders and Youth: NWMO. (n.d.). Retrieved from https://www.nwmo.ca/en/ABOUT-US/How-We-re-Governed/Council-of-Elders .
- Indigenous Engagement: NWMO. (n.d.). Retrieved from https://www.nwmo.ca/en/A-Safe-Approach/About-the-Project/Working-in-Partnership/Engaging-With-People/Indigenous-Engagement .
- Natural Resources Canada. (2019, November 18). Retrieved from https://www.nrcan.gc.ca/home .
- Indigenous Knowledge: NWMO. (n.d.). Retrieved from https://www.nwmo.ca/en/Site-selection/About-the-Process/Indigenous-Knowledge .
Other websites
- Heritage Futures/SKB. Nuclear waste must be managed for thousands of years. What has heritage to learn and share on long term preservation? (n.d.). Retrieved from https://heritage-futures.org/uncertainty/nuclear-waste-management /.
Feature image credit: https://www.nwmo.ca/en/More-information/News-and-Activities/2018/07/16/16/47/The-NWMO-issues-reconciliation-statement-collaboratively-with-Indigenous-Elders-and-youth
What happens to nuclear waste under Peter Dutton's Coalition plan to build seven nuclear power reactors?
Regardless of whether the Coalition's nuclear vision comes to fruition, the clock is already ticking on plans to deal with nuclear waste.
Australia currently produces a relatively small amount of radioactive waste due to its limited nuclear industry, with just one reactor used for research and medical purposes, located at Lucas Heights in Sydney.
Low-level waste in the form of medical equipment, clothing and face masks is stored at around 100 locations despite plans for decades to build one centralised facility.
"While Australia has no nuclear power producing electricity, it does have well-developed usage of radioisotopes in medicine, research and industry," a spokesperson for nuclear industry organisation the World Nuclear Association said.
"Each year Australia produces about 45 cubic metres of radioactive waste arising from these uses and from the manufacture of the isotopes."
This amounts to about 40 square metres of low-level waste and 5 square metres of intermediate waste, while the UK and France by comparison each produce about 25,000 cubic metres of low-level waste annually.
But of greater concern is the intermediate and high-level waste that will be produced by the seven nuclear reactors the Coalition plans to get up and running in Australia by 2050.
Peter Dutton in announcing the Coalition's nuclear plan this week used a previously heard line that one standard-sized reactor produces just a handful of nuclear waste each year.
"If you look at a 450 megawatt reactor, it produces waste equivalent to the size of a can of Coke each year," Mr Dutton said.
"It's stored on site under our proposal, and then at the end of the life of that asset, it's moved to a permanent home.
"Our argument is that should be where the government decides for the waste from the submarines to be stored."
Simon Holmes a Court is well known for his role as convenor of Climate 200 and the crowdfunded initiative's successful funding of so-called "Teal" candidates at the last federal election, but he is also a director of the Smart Energy Council and The Superpower Institute dedicated to decarbonising the economy.
He said the Coke can comment greatly underestimates the amount reactors generate.
"Even the small modular reactors would be 2,000 times as much, and that is just high-level radioactive waste alone," he said.
"It is a lot more than he says but we do have a problem in Australia.
"We have tried for 20 years or more to build a low-level radioactive waste facility and it keeps meeting all sorts of opposition."
He is referring to the creation of a national nuclear storage facility that got to the point of a government purchase of a 221-hectare property near the town of Kimba, west of Whyalla in South Australia, but this was scuttled in July last year when traditional owners took the matter to court and won.
The Barngarla people have a sacred site for women near Kimba which they feared the facility would destroy, and this effectively stopped it.
The waste storage site will be needed for waste from the AUKUS submarines regardless of the Coalition's nuclear energy plans.
The AUKUS deal is bipartisan, so any change of government is unlikely to scuttle it.
Griffith University emeritus professor and energy specialist Ian Lowe told The Conversation that Australia will have to manage high-level radioactive waste when the submarines are decommissioned in 30 years time.
"So, when our first three subs are at the end of their lives – which, according to Defence Minister Richard Marles, will be in about 30 years time – we will have 600 kilograms of so-called 'spent fuel' and potentially tonnes of irradiated material from the reactor and its protective walls," he said.
"Because the fuel is weapons-grade material, it will need military-scale security," he said.
"This waste needs to be permanently isolated from ecosystems and human society, given it will take tens of thousands of years for the radiation to decay to safe levels."
Currently Australia's intermediate level nuclear waste generated at the Lucas Height reactor is taken overseas for processing then returned to Australia for storage.
Remaining unused uranium is removed from the fuel rods with the leftover radioactive waste broken up and mixed with molten glass, then solidified in steel canisters.
The last time this happened, in March 2022, it involved a shipment of radioactive waste brought back to Lucas Heights via a high security operation at Port Kembla in Wollongong.
"Four of those canisters, each containing 500 kilograms of vitrified waste that is radiologically equivalent to 114 rods sent to the UK in a shipment in 1996, were received back from the UK," according to a statement from Australia's Nuclear Science and Technology Organisation (ANSTO).
It was logistically a major operation carried out in relative secrecy in the middle of the night with confirmation only occurring afterwards.
Such shipments only tend to occur about once every 10 years, but this all could start to change if and when Australia moves towards embracing a larger role for nuclear.
Want to know more about the nuclear power announcement? Send us your questions and we'll try to answer them as part of our coverage.
- X (formerly Twitter)
Related Stories
Peter dutton has placed a target on his back. is his nuclear policy cunning genius or political self-destruction.
Peter Dutton has announced the sites for proposed nuclear reactors. Here's what comes next
- Alternative Energy
- Energy Industry
- Federal - State Issues
- Federal Government
- Government and Politics
- Lucas Heights
- Nuclear Energy
- Nuclear Issues
- Port Kembla
- Recycling and Waste Management
A comprehensive decision-making framework to configure a viable healthcare waste management system considering pollution risk: a case study
- Original Paper
- Published: 17 June 2024
Cite this article
- Parya Koohkan 1 ,
- Ebrahim Asadi-Gangraj 1 &
- Sina Nayeri 2
29 Accesses
Explore all metrics
In recent years, designing an efficient healthcare waste management system is known as one of the leading environmental issues and it is one of the critical challenges for the related managers. In this regard, due to the importance of proper management of this waste and despite the existence of a significant number of studies, some gaps are still observed in this field. In this regard, to bridge these gaps, this study develops a decision-making framework to configure a healthcare waste chain by considering the viability dimensions and the Internet of Things application. To do this, a scenario-based multi-objective model is proposed that simultaneously considers the sustainability, digitalization, agility, and resiliency aspects. In this study, to measure the pollution risk, the failure mode and effects analysis method is employed. Then, the proposed mathematical model is solved using the Chebyshev multi-choice goal programming with utility function. Overall, the main contribution of this work is to design an Internet of Things-enabled healthcare waste chain considering the viability dimensions and pollution risk for the first time. The results showed that employing the Internet of Things can significantly reduce the pollution risk. Also, according to the achieved results, the visibility of the waste chain was significantly enhanced when the blockchain-based information-sharing system was established. Moreover, the results of the sensitivity show that an increase in the rate of disruption leads to a 28% increase in total costs, a 15% increase in environmental impacts, and a 22% decrease in visibility.
Graphical abstract
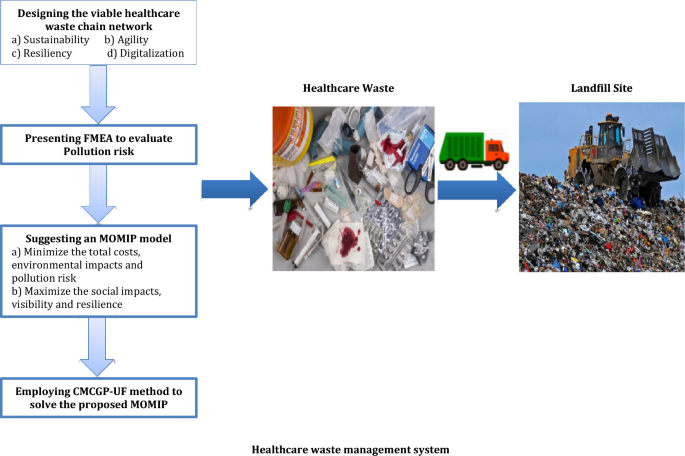
A schematic from the proposed viable waste chain
This is a preview of subscription content, log in via an institution to check access.
Access this article
Price includes VAT (Russian Federation)
Instant access to the full article PDF.
Rent this article via DeepDyve
Institutional subscriptions
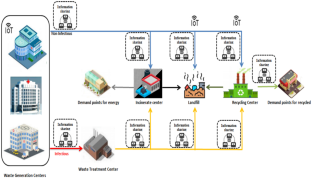
Similar content being viewed by others
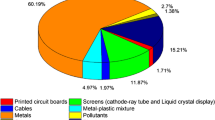
A review of the recent development, challenges, and opportunities of electronic waste (e-waste)
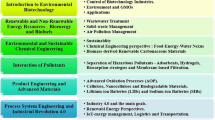
Recent advances in green technology and Industrial Revolution 4.0 for a sustainable future
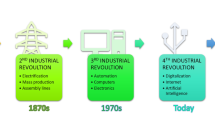
Industry 5.0 or industry 4.0S? Introduction to industry 4.0 and a peek into the prospective industry 5.0 technologies
Availability of data and material.
Not applicable.
Abdolazimi O, Ma J, Shishebori D, Alimohammadi Ardakani M, Erfan Masaeli S (2023) A multi-layer blood supply chain configuration and optimization under uncertainty in COVID-19 pandemic. Comput Ind Eng 182:109441. https://doi.org/10.1016/j.cie.2023.109441
Article Google Scholar
Arabsheybani A, Paydar MM, Safaei AS (2018) An integrated fuzzy MOORA method and FMEA technique for sustainable supplier selection considering quantity discounts and supplier’s risk. J Clean Prod 190:577–591
Babaee Tirkolaee E, Aydın NS (2021) A sustainable medical waste collection and transportation model for pandemics. Waste Manag Res 39:34–44
Article CAS Google Scholar
Banguera L, Sepúlveda JM, Fuertes G, Carrasco R, Vargas M (2017) Reverse and inverse logistic models for solid waste management. South African J Ind Eng 28(4):120–132. https://doi.org/10.7166/28-4-1701
Cao C, Xie Y, Liu Y, Liu J, Zhang F (2023) Two-phase COVID-19 medical waste transport optimisation considering sustainability and infection probability. J Clean Prod 389:135985. https://doi.org/10.1016/j.jclepro.2023.135985
Cardoso SR, Barbosa-Póvoa AP, Relvas S, Novais AQ (2015) Resilience metrics in the assessment of complex supply-chains performance operating under demand uncertainty. Omega 56:53–73
Dixit A, Dutta P (2024) Critical success factors for the adoption of circular economy in sustainable healthcare waste management. Clean Technol Environ Policy. https://doi.org/10.1007/s10098-023-02712-y
Eren E, Rıfat Tuzkaya U (2021) Safe distance-based vehicle routing problem: medical waste collection case study in COVID-19 pandemic. Comput Ind Eng 157:107328. https://doi.org/10.1016/j.cie.2021.107328
Goodarzian F, Ghasemi P, Gunasekaran A, Labib A (2024) A fuzzy sustainable model for COVID-19 medical waste supply chain network. Fuzzy Optim Decis Mak 23(1):93–127. https://doi.org/10.1007/s10700-023-09412-8
Ho CC, Liao CJ (2011) The use of failure mode and effects analysis to construct an effective disposal and prevention mechanism for infectious hospital waste. Waste Manag 31(12):2631–2637. https://doi.org/10.1016/j.wasman.2011.07.011
Homayouni Z, Pishvaee MS (2020) A bi-objective robust optimization model for hazardous hospital waste collection and disposal network design problem. J Mater Cycles Waste Manag 22(6):1965–1984. https://doi.org/10.1007/s10163-020-01081-8
Ivanov D (2022) Viable supply chain model: integrating agility, resilience and sustainability perspectives—lessons from and thinking beyond the COVID-19 pandemic. Annals of Oper Res 319(1):1411–1431
Kannan D, Khademolqorani S, Janatyan N, Alavi S (2024) Smart waste management 4.0: the transition from a systematic review to an integrated framework. Waste Manag 174:1–14
Kargar S, Paydar MM, Safaei AS (2020) A reverse supply chain for medical waste: A case study in Babol healthcare sector. Waste Manag 113:197–209
Karimi H, Wassan N, Ehsani B, Tavakkoli-Moghaddam R, Ghodratnama A (2024) Optimizing COVID-19 medical waste management using goal and robust possibilistic programming. Eng Appl Artif Intell 131:107838
Kaushal R, Rohit, Dhaka AK (2024) A comprehensive review of the application of plasma gasification technology in circumventing the medical waste in a post-COVID-19 scenario. Biomass Convers Biorefin 14(2):1427–1442. https://doi.org/10.1007/s13399-022-02434-z
Kim Y, Chen YS, Linderman K (2015) Supply network disruption and resilience: a network structural perspective. J Oper Manag 33–34:43–59. https://doi.org/10.1016/j.jom.2014.10.006
Kordi G, Hasanzadeh-Moghimi P, Paydar MM, Asadi-Gangraj E (2023) A multi-objective location-routing model for dental waste considering environmental factors. Annals of Oper Res 328(1):755–792. https://doi.org/10.1007/s10479-022-04794-1
Liao CJ, Ho CC (2014) Risk management for outsourcing biomedical waste disposal—using the failure mode and effects analysis. Waste Manag 34(7):1324–1329. https://doi.org/10.1016/j.wasman.2014.03.007
Liu H, Deng X, Jiang W (2017) Risk evaluation in failure mode and effects analysis using fuzzy measure and fuzzy integral. Symmetry 9(8):1195–1207. https://doi.org/10.3390/sym9080162
Lotfi R, Kargar B, Gharehbaghi A, Weber GW (2021) Viable medical waste chain network design by considering risk and robustness. Environ Sci Pollut Res. https://doi.org/10.1007/s11356-021-16727-9
Lotfi R, Kargar B, Gharehbaghi A, Weber GW (2022) Viable medical waste chain network design by considering risk and robustness. Environ Sci Pollut Res 29(53):79702–79717. https://doi.org/10.1007/s11356-021-16727-9
Lotfi R, Mehrjardi MS, MohajerAnsari P, Zolfaqari F, Afshar M (2023) Antifragile, sustainable, and agile supply chain network design by considering resiliency, robustness, risk, and environmental requirements. Environ Sci Pollut Res 30(48):106442–106459. https://doi.org/10.1007/s11356-023-29488-4
Lotfi R, Hazrati R, Aghakhani S, Afshar M, Amra M, Ali SS (2024) A data-driven robust optimization in viable supply chain network design by considering open innovation and blockchain technology. J Cleaner Prod 436:140369
Lotfi R, Mardani N, Alic SS, Maryam S (2024) A robust and risk-averse medical waste chain network design by considering viability requirements. RAIRO-Oper Res 58(2):1473–1497
Mamashli Z, Nayeri S, Tavakkoli-Moghaddam R, Sazvar Z, Javadian N (2021) Designing a sustainable–resilient disaster waste management system under hybrid uncertainty: a case study. Eng Appl Artif Intell 106:104459
Mim KN, Alam MZ, Nowshin N (2024) Scenario of medical waste management in bangladesh during Covid-19. Lecture Notes in Civil Eng 368:189–199. https://doi.org/10.1007/978-981-99-3826-1_16
Mohamed NH, Khan S, Jagtap S (2023) Modernizing medical waste management: unleashing the power of the Internet of Things (IoT). Sustainability (switzerland) 15(13):9909. https://doi.org/10.3390/su15139909
Mohamed NH, Khan S, Jagtap S (2022). Towards digitalization of Malaysian Medical Facilities Waste Management .
Namdar J, Torabi SA, Sahebjamnia N, Nilkanth Pradhan N (2021) Business continuity-inspired resilient supply chain network design. Int J Prod Res 59(5):1331–1367
Nayeri S, Torabi SA, Tavakoli M, Sazvar Z (2021) A multi-objective fuzzy robust stochastic model for designing a sustainable-resilient-responsive supply chain network. J Cleaner Prod 311:127691
Nayeri S, Khoei MA, Rouhani-Tazangi MR, GhanavatiNejad M, Rahmani M, Tirkolaee EB (2023) A data-driven model for sustainable and resilient supplier selection and order allocation problem in a responsive supply chain: a case study of healthcare system. Eng Appl Artif Intell 124:106511
Negarandeh R, Tajdin A (2022) A robust fuzzy multi-objective programming model to design a sustainable hospital waste management network considering resiliency and uncertainty: a case study. Waste Manag Res 40(4):439–457
Palita FB, Purnaweni H, Luqman Y (2024) Evaluation of solid medical waste management system in Undata Regional General Hospital, Palu city, Central Sulawesi Province. Indonesia J Bioresour Environ Sci 3(2):70–77. https://doi.org/10.61435/jbes.2024.19923
Sanito RC, Mujiyanti DR, You SJ, Wang YF (2024) A review on medical waste treatment in COVID-19 pandemics: technologies, managements and future strategies. J Waste Manag Assoc 74(2):72–99. https://doi.org/10.1080/10962247.2023.2282011
Shekdar AV (2009) Sustainable solid waste management: an integrated approach for Asian countries. Waste Manag 29(4):1438–1448
Subramanian, N, Chaudhuri A, Kayıkcı Y (2020). Blockchain and Supply Chain Logistics: Evolutionary Case Studies . Springer Nature.
Suksee S, Sindhuchao S (2021) Grasp with alns for solving the location routing problem of infectious waste collection in the Northeast of Thailand. Int J Ind Eng Computations 12(3):305–320. https://doi.org/10.5267/j.ijiec.2021.2.001
Taheri F, Moghaddam BF (2022) A heuristic-based hybrid algorithm to configure a sustainable supply chain network for medical devices considering information-sharing systems. Environ Sci Pollut Res. https://doi.org/10.1007/s11356-022-22147-0
Tirkolaee EB, Abbasian P, Weber G-W (2021) Sustainable fuzzy multi-trip location-routing problem for medical waste management during the COVID-19 outbreak. Sci Total Environ 756:143607
Torkayesh AE, Vandchali HR, Tirkolaee EB (2021) Multi-objective optimization for healthcare waste management network design with sustainability perspective. Sustainability 13(15):8279
Wahyati Yustina E, Nurmardiansyah E, Saraswati R, Elwina Simandjuntak M (2024) How to Optimize Local Government Supervision of Medical Waste Management in Healthcare Facilities KnE Social Sciences , https://doi.org/10.18502/kss.v8i21.14709
Wang N, Cui W, Zhang M, Jiang Q (2023) Routing optimization for medical waste collection considering infectious risk and multiple disposal centers. Expert Syst Appl 234:121035. https://doi.org/10.1016/j.eswa.2023.121035
Wang Q, Zhang M, Li R (2024) Does medical waste research during COVID-19 meet the challenge induced by the pandemic to waste management? Waste Manag Res 42(3):244–259. https://doi.org/10.1177/0734242X231178226
Wu H, Yang B, Tao F (2020) Optimization of vehicle routing for waste collection and transportation. Int J Environ Res Public Health 17(14):1–26. https://doi.org/10.3390/ijerph17144963
Zarrinpoor N (2023) A sustainable medical waste management system design in the face of uncertainty and risk during COVID-19. Fuzzy Optim Decis Mak 22(3):519–554. https://doi.org/10.1007/s10700-022-09401-3
Zhao J, Wu B, Ke GY (2021) A bi-objective robust optimization approach for the management of infectious wastes with demand uncertainty during a pandemic. J Cleaner Prod 314:127922
Download references
No funding was received.
Author information
Authors and affiliations.
Babol Noshirvani University of Technology, Babol, Iran
Parya Koohkan & Ebrahim Asadi-Gangraj
Department of Industrial Engineering, University of Tehran, Tehran, Iran
Sina Nayeri
You can also search for this author in PubMed Google Scholar
Contributions
Parya Koohkan contributed to conceptualization, methodology, software, original draft preparation, visualization. Ebrahim Asadi-Gangraj contributed to Supervision, investigation, writing-reviewing and editing, Sina Nayeri helped in software, methodology, validation, writing-reviewing and editing.
Corresponding author
Correspondence to Ebrahim Asadi-Gangraj .
Ethics declarations
Conflict of interest.
The authors declare that there is no conflict of interest.
Ethics approval and consent to participate
Consent for publication, additional information, publisher's note.
Springer Nature remains neutral with regard to jurisdictional claims in published maps and institutional affiliations.
Applying FMEA for calculating the pollution risk
This study employed FMEA analysis to measure the pollution risk level associated with various factors, including overflowing garbage bins. The study also aimed to demonstrate the advantages of utilizing (IoT) technology by comparing the pollution risk with and without the use of IoT. The team for the FMEA consisted of 3 experienced experts to consider the failure modes of each waste generation facility. The data for evaluate failure modes for each risk factor, in different points of waste generation based on experts' opinions, are stated in Tables 9 and 10 . It should be noted that each FMEA team member is assumed to be equally important. In first, the team members collected the evaluation data based on Table 9 and Table 10 for Eq. ( 34 ). Then, the results of the collection of evaluation information for 3 failure modes are presented in Tables 11 and 12 . Finally, RPNs of waste generation locations, according to Eq. ( 34 ), are shown in the last column of Tables 11 and 12 . After comparing the RPN numbers in Table A.10 , it was determined that waste generation center #1 poses the highest pollution risk, while waste generation center #3 poses the least pollution risk. Also by comparing Tables 11 and Table 12 , it can be concluded that the RPN has a lower value when IoT is established. This implies that establishing the IoT system will significantly reduce the pollution risk.
Rights and permissions
Springer Nature or its licensor (e.g. a society or other partner) holds exclusive rights to this article under a publishing agreement with the author(s) or other rightsholder(s); author self-archiving of the accepted manuscript version of this article is solely governed by the terms of such publishing agreement and applicable law.
Reprints and permissions
About this article
Koohkan, P., Asadi-Gangraj, E. & Nayeri, S. A comprehensive decision-making framework to configure a viable healthcare waste management system considering pollution risk: a case study. Clean Techn Environ Policy (2024). https://doi.org/10.1007/s10098-024-02905-z
Download citation
Received : 24 March 2024
Accepted : 26 May 2024
Published : 17 June 2024
DOI : https://doi.org/10.1007/s10098-024-02905-z
Share this article
Anyone you share the following link with will be able to read this content:
Sorry, a shareable link is not currently available for this article.
Provided by the Springer Nature SharedIt content-sharing initiative
- Waste management
- Healthcare waste chain; Viability
- Internet of Things; FMEA
- Multi-objective decision-making
- Find a journal
- Publish with us
- Track your research

IMAGES
VIDEO
COMMENTS
Long-term, sustainable solutions to radioactive waste management. Scientific Reports 14, Article number: 5907 ( 2024 ) Cite this article. Nuclear power plays a pivotal role in ensuring a scalable ...
Three case studies on radioactive waste. 9 December 2021. These are case studies in a larger report on radioactive waste and transparency, currently under preparation for the Euratom EURAD programme by Nuclear Transparency Watch. Publication was expected in October 2021.
and proliferation of nuclear waste. Three major Spent Nuclear Fuel management approaches i.e. SNF reprocessing, deep geological repository and SNF export where studied together with five factors that affect policies on nuclear waste management in the Nigerian context. At the end of the study SNF export was found to more suitable than the other ...
In terms of overall volume, around 95% of existing radioactive waste has very low level (VLLW) or low-level (LLW) radioactivity, while about 4% is intermediate level waste (ILW) and less than 1% is high-level waste (HLW). Since the start of nuclear electricity production in 1954 to the end of 2016, some 390,000 tonnes of spent fuel were generated.
The study concludes that, overall, small modular designs are inferior to conventional reactors with respect to radioactive waste generation, management requirements, and disposal options.
The reviewed papers presented here provide a general overview of worldwide radioactive waste-related studies conducted in 2019. The current review includes studies related to safety assessments, decommission and decontamination of nuclear facilities, fusion facilities, and transportation. Further, the review highlights radioactive wastewater ...
Description. Nuclear Decommissioning Case Studies: Characterization, Waste Management, Reuse and Recycle, Author's Statement on the Sustainability of Nuclear Decommissioning, Volume Six presents a selection of global case studies that focus on a range of technologies for the decontamination, dismantling, spent fuel treatment and recycling of ...
"Our results show that most small modular reactor designs will actually increase the volume of nuclear waste in need of management and disposal, by factors of 2 to 30 for the reactors in our case study," said study lead author Lindsay Krall, a former MacArthur Postdoctoral Fellow at Stanford University's Center for International Security ...
In 1987, the Nuclear Waste Policy Act Amendments (NWPAA) assigned Yucca Mountain, adjacent to the Nevada Test Site, as the permanent storage site for nuclear waste in the United States (Tamayo et al. 2020). The radioactive waste was stored by burying radioactive waste deep into the ground after it was completely sealed in a dry cask (Kyne and ...
Finally, case studies for the applications of different technologies in the treatment, storage, and disposal of liquid and solid wastes will be presented and analyzed to have insights into the lessons learned from these practices. ... Nuclear Waste Management Facilities is a crucial tool needed to implement the safest and most environmentally ...
disposal programme and establishing funding mechanisms. It is applicable to all waste categories and both near surface and geological disposal, and contains relevant examples and case studies from national programmes (Fig. 1). Design Principles and Approaches for Radioactive Waste Repositories (IAEA Nuclear Energy Series No. NW-T-1.27 ...
Case Studies 3-4: Two Low-Level Waste Case Studies from Canada. Mr. Garamszeghy's presentation was split into three parts: background on Canadian nuclear regulation and management, a case study on the Port Hope Area Initiative (PHAI), and a case study on the Deep Geological Repository for low- and intermediate-level wastes.
Addressing Challenges in Managing Radioactive Waste from Past Activities. IAEA-TECDOC -2039. English IAEA-TECDOC-2039 ¦ 978-92--102724-5. 23 figures ¦ € 30.00 ¦ Date published: 2024. Download PDF (2.8 MB)
Nuclear Decommissioning Case Studies: Characterization, Waste Management, Reuse and Recycle, Author's Statement on the Sustainability of Nuclear Decommissioning, Volume Six presents a selection of global case studies that focus on a range of technologies for the decontamination, dismantling, spent fuel treatment and recycling of nuclear decommissioning.
Using a qualitative approach combining policy analysis and case studies, we examine this liminality and the consequent emergence of a new political economy of spent fuel management. Our findings indicate a series of regulatory, organizational and spatial 'fixes' have recently emerged to capitalize on the liminality of nuclear waste ...
Case Studies 3-4: Two Low-Level Waste Case Studies from Canada. Mr. Garamszeghy's presentation was split into three parts: background on Canadian nuclear regulation and management, a case study on the Port Hope Area Initiative (PHAI), and a case study on the Deep Geological Repository for low- and intermediate-level wastes.
The fundamental principles of nuclear waste management are: to ensure the. generation of nuclear waste is kept to a minimum; to protect human health and. the environment; and to protect future ...
by Madeleine Jennewein figures by Rebecca Senft. Across the United States, nuclear waste is accumulating in poorly maintained piles. 90,000 metric tons of nuclear waste requiring disposal are currently in temporary storage. The United States, however, has yet to construct a long-term storage solution for this waste, leaving the nuclear material vulnerable to extreme weather events such as ...
January 7-8, 2008, Vanderbilt University, Nashville, TN Watch video and listen to podcasts from "Uncertainty in Long Term Planning-Nuclear Waste Management, A Case Study." The Jan. 7-8, 2008, symposium and celebration was held in honor Frank L. Parker, Distinguished Professor of Water Resources and Environmental Engineering at Vanderbilt University. The conditions of nuclear waste ...
The study performed by Radioactive Waste Management Ltd. [57] on the radiological impact of nuclear wastes disposed in a Geological Disposal Facility (GDF) reports similar results: the radiological impact from ILW is larger than that from HLW, and I129 and Cl36 represent the primary sources of impact [57]. The two radiological categories ...
Comparative case studies are useful analytical tools when evaluating multiple programs with different outcomes such as in the case of nuclear waste disposal in the US (Yin, 2014). A limitation of the case study approach is external validity which focusses on the generalizability of the findings to other cases.
2008. Traditional Knowledge Project (Personal experiences in Ontario's DGR to educate the Indigenous peoples about the nuclear waste management process and in Mackenzie River to enable the technical staff to value Indigenous peoples; and a Workshop) 2009. Memorandum of Understanding between Natural Resources Canada (NRCan) and NWMO.
The waste storage site will be needed for waste from the AUKUS submarines regardless of the Coalition's nuclear energy plans. The AUKUS deal is bipartisan, so any change of government is unlikely ...
In recent years, designing an efficient healthcare waste management system is known as one of the leading environmental issues and it is one of the critical challenges for the related managers. In this regard, due to the importance of proper management of this waste and despite the existence of a significant number of studies, some gaps are still observed in this field. In this regard, to ...
Although the focus was on decommissioning, nuclear waste management repeatedly surfaced in all case study countries. Access to waste disposal emerged as a critical issue affecting decommissioning in terms of financing, organization, regulation, and production. The availability of storage and disposal for LLW, ILW, and HLW significantly impacts ...